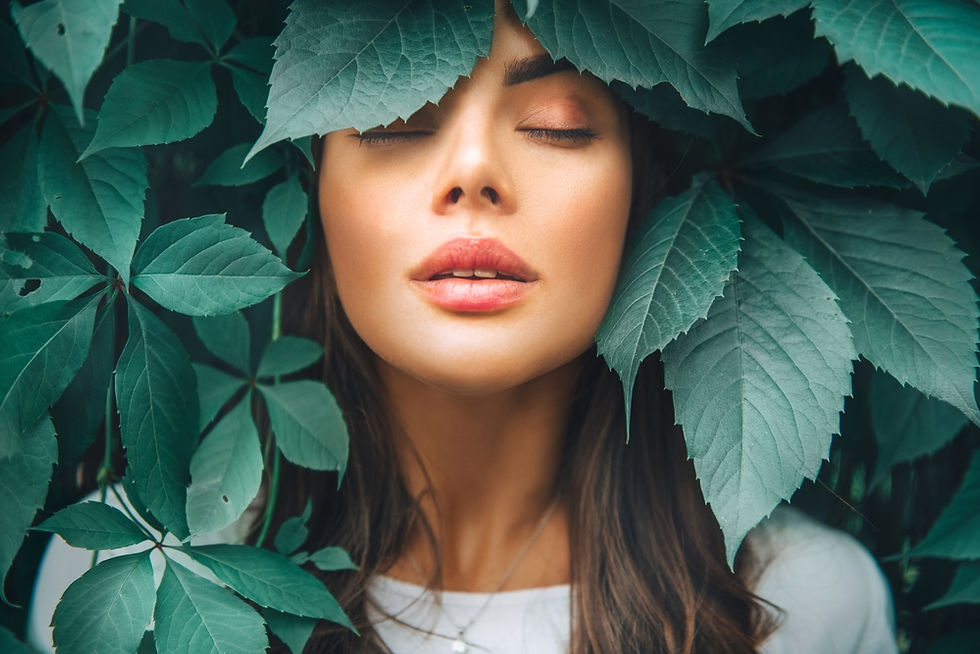
We answer four breathing questions from clinical and optimal performance professionals.
What is the physiology of sighing? Sighing represents a sophisticated physiological mechanism that serves both respiratory and emotional functions. Li et al. (2016) revealed that this behavior originates in specialized neural circuits within the pre-Bötzinger complex and retrotrapezoid nucleus (RTN) of the brainstem, areas crucial for respiratory rhythm regulation.

Caption: OpenStax College - Anatomy & Physiology, Connexions Web site. http://cnx.org/content/col11496/1.6/, Jun 19, 2013., CC BY 3.0, https://commons.wikimedia.org/w/index.php?curid=30148399
These regions respond to specific neuropeptides, particularly bombesin-like peptides, including neuromedin B and gastrin-releasing peptides, which trigger the characteristic sighing pattern by activating pre-Bötzinger complex neurons.
The respiratory mechanics of sighing involve a distinctive pattern: two deep inhalations followed by an extended, slow exhalation. According to Cherniack et al. (2017), this pattern serves a vital function in maintaining lung health by recruiting potentially collapsed alveoli, thereby preserving normal lung compliance and preventing atelectasis. This mechanism becomes particularly important during periods of prolonged shallow breathing.
Komeili et al. (2020) documented the cardiovascular effects of sighing, noting that the slow exhalation phase activates the parasympathetic nervous system, potentially lowering both heart rate and blood pressure. This physiological response contributes to the calming effect often associated with sighing.
The emotional aspects of sighing are equally significant. Vlemincx et al. (2013) proposed that sighing functions as a "reset" mechanism for both physiological and emotional states. During relief, frustration, or sadness, the limbic system, particularly the amygdala, communicates with brainstem respiratory centers to modulate sighing patterns in response to emotional arousal.
From an adaptive perspective, sighing serves dual functions in maintaining homeostasis. First, it optimizes respiratory function by increasing tidal volume and improving blood gas levels. Second, the extended exhalation stimulates vagal nerve activity, promoting a calming effect on the nervous system. This combination of physiological and emotional regulation highlights sighing's role as a crucial adaptive response to physical and psychological stressors.
What is the physiology of yawning?
Yawning, a fascinating physiological behavior observed across vertebrates, involves intricate neural and muscular mechanisms that scientists are still working to fully understand. While researchers debate its precise function, evidence suggests yawning plays important roles in arousal, temperature regulation, and social interaction.
The neural control of yawning centers in the brainstem, specifically within the hypothalamus's paraventricular nucleus (PVN). Sanna et al. (2012) demonstrated that oxytocinergic neurons in the PVN are crucial for initiating yawns. The behavior depends on a complex interplay of neurotransmitters, with Collins and Eguibar (2010) showing that increased dopaminergic activity correlates with more frequent yawning. Other key neurotransmitters include oxytocin, acetylcholine, and various excitatory amino acids.
When a yawn occurs, it triggers a coordinated sequence of muscle activations. The craniofacial muscles, including the masseter for jaw movement and orbicularis oris controlling the mouth, contract to create the characteristic wide-mouthed expression. Simultaneously, the diaphragm and respiratory muscles engage in a distinctive pattern: a deep inhalation, followed by a momentary pause, and finally, a slower exhalation.
These muscular actions produce significant cardiorespiratory effects. As Gallup and Gallup (2007) noted, yawning increases airflow through the respiratory system, potentially enhancing blood oxygenation and cooling blood flowing through the carotid artery. The autonomic nervous system activation during yawning also causes a temporary elevation in heart rate.
The thermoregulatory hypothesis, advanced by Gallup and Gallup (2007), suggests that yawning helps regulate brain temperature. According to this theory, the intake of cool air during a yawn promotes cerebral blood flow and heat exchange, effectively cooling the brain. This mechanism may explain why we often yawn when tired or overheated.
The social dimension of yawning is particularly intriguing. Platek et al. (2005) explored how yawning's contagious nature likely stems from mirror neuron activity, linking this behavior to social bonding and empathy in humans and other animals. This social aspect underscores yawning's evolution from a simple physiological response to a complex behavior with important social interaction and communication implications.

How does using a CPAP machine influence breathing at night?
A Continuous Positive Airway Pressure (CPAP) machine operates through constant pressurized airflow to maintain open airways during sleep. As documented by Sullivan et al. (1981), this pressurized air prevents the collapse of soft tissues in the pharynx, including the soft palate, tongue, and pharyngeal walls, effectively eliminating apneic events and reducing snoring.
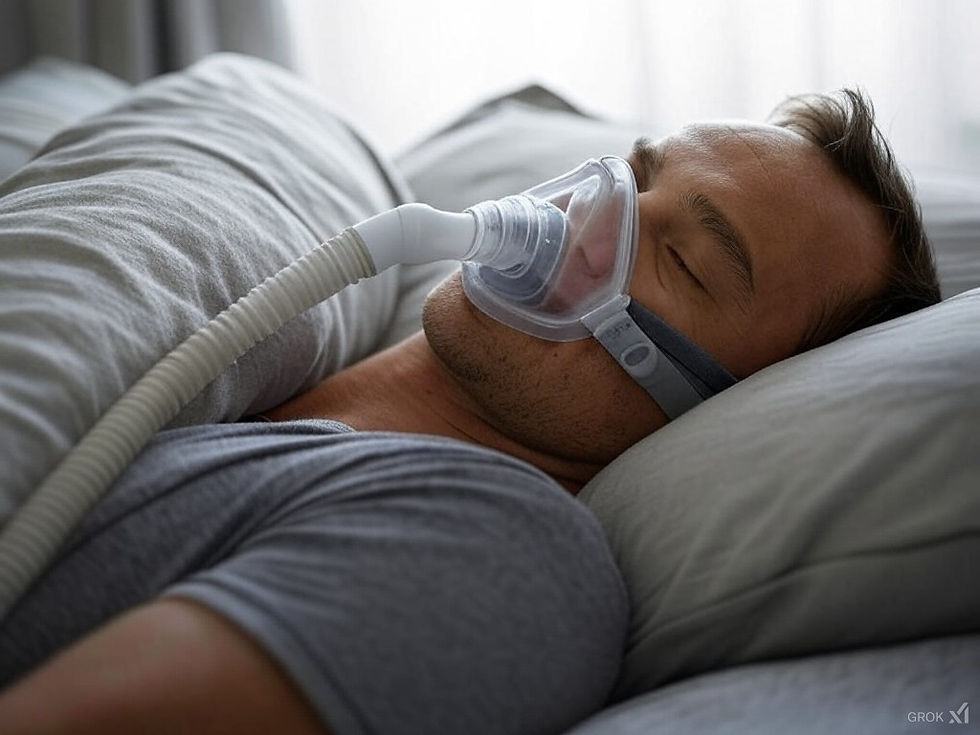
The restoration of normal breathing patterns through CPAP therapy produces significant physiological benefits. Malhotra and White (2002) demonstrated that improved gas exchange reduces hypoxemia and hypercapnia, enhancing oxygen delivery to tissues and lowering the risk of cardiovascular and neurocognitive complications associated with untreated obstructive sleep apnea (OSA). Additionally, CPAP reduces respiratory effort by maintaining airway patency. Eckert et al. (2007) found that this stabilization of breathing patterns prevents oscillations in blood gases that can lead to central apnea or Cheyne-Stokes respiration.
Research by Somers et al. (2008) revealed that CPAP therapy reduces sympathetic nervous system activation by preventing intermittent hypoxia and promoting cardiovascular health through decreased blood pressure and heart rate. Epstein et al. (2009) further documented how CPAP improves sleep quality by reducing sleep fragmentation and promoting deeper sleep stages, alleviating common OSA symptoms like daytime sleepiness and cognitive impairment.
However, Weaver and Grunstein (2008) noted that CPAP effectiveness heavily depends on patient compliance. Poor mask fit, physical discomfort, and claustrophobia can significantly impact adherence and diminish therapeutic benefits. This highlights the importance of proper device fitting and patient education in successful CPAP therapy.
What is the evidence supporting the efficacy of mouth or nasal taping during sleep?
Research on mouth and nasal taping during sleep reveals potential benefits and risks. Li et al. (2015) documented how nasal breathing improves oxygen uptake through better filtration, humidification, and warming of inhaled air while reducing oral dryness and promoting dental health.

Studies specifically examining mouth taping's effectiveness show promising initial results. Meen et al. (2021) found reduced snoring intensity and frequency among participants using mouth tape, attributing improvements to enhanced nasal airflow. This aligns with Randerath et al.'s (2018) findings that nasal breathing reduces inspiratory effort and stabilizes the upper airway.
Evidence suggests limited applications for obstructive sleep apnea (OSA). Stokes et al. (2019) demonstrated that while mouth taping improved CPAP compliance by reducing mouth leaks, it proved insufficient as a standalone OSA treatment. Their research also highlighted potential risks, including skin irritation, breathing obstruction during nasal congestion, and psychological discomfort.
Psychological benefits reported in Meen et al.'s (2021) work, including improved sleep quality and reduced nighttime awakenings, remain largely anecdotal. Current research limitations include small sample sizes, observational study designs, and reliance on subjective reporting. Long-term effects and impacts on individuals with comorbidities remain poorly understood.
While mouth taping shows promise for mild OSA and habitual snoring, its broader therapeutic value requires validation through larger, randomized controlled trials. Current evidence suggests cautious implementation, particularly for individuals with moderate to severe OSA or underlying respiratory conditions.
What are the health risks of patients with untreated sleep apnea?
Untreated obstructive sleep apnea (OSA) triggers cascading health effects through repeated airway obstruction, oxygen deprivation and disrupted sleep. Pedrosa et al. (2011) documented OSA's strong connection to resistant hypertension, while Somers et al. (2008) showed increased risks of arrhythmias, heart attacks, and heart failure due to cardiovascular stress. Redline et al. (2010) found higher stroke risks stemming from endothelial dysfunction and blood clotting abnormalities.
Metabolically, Reutrakul and Mokhlesi (2017) identified OSA as an independent risk factor for insulin resistance and type 2 diabetes, while Li et al. (2014) demonstrated its disruption of lipid metabolism. Cognitive impacts are equally severe, with Ju et al. (2019) linking severe OSA to increased dementia risk, including Alzheimer's disease. Harris et al. (2009) documented its contribution to mood disorders through sleep disruption and oxygen deprivation.
Boudewyns et al. (2000) showed how hypoxic pulmonary vasoconstriction in OSA can lead to pulmonary hypertension, which is particularly dangerous for those with existing lung conditions. Tregear et al. (2009) found that resulting daytime sleepiness significantly increases accident risks. Young et al. (2008) demonstrated higher all-cause mortality rates in severe untreated cases, particularly from cardiovascular events. Beyond these direct effects, Besedovsky et al. (2012) documented how chronic sleep disruption compromises immune function, increasing infection susceptibility and impairing inflammatory responses.
Breathing Glossary
atelectasis: a condition where the alveoli in the lungs collapse, reducing gas exchange and lung compliance.
bombesin-like peptides: neurotransmitters, including neuromedin B and gastrin-releasing peptides, that regulate specific brainstem respiratory mechanisms.
Cheyne-Stokes respiration: a cyclic pattern of breathing characterized by gradual increases and decreases in respiration followed by apnea.
CPAP (Continuous Positive Airway Pressure): a device delivering constant pressurized air to maintain open airways during sleep.
endothelial dysfunction: impaired blood vessel lining function, often linked to cardiovascular diseases and sleep apnea.
hypoxic pulmonary vasoconstriction: a process in which blood vessels in the lungs constrict in response to low oxygen levels.
mirror neurons: neurons that fire when an action is performed and when it is observed in others, linked to empathy and social behaviors.
neuromedin B: a peptide neurotransmitter involved in respiratory rhythm and sigh regulation.
Parasympathetic nervous system: The division of the autonomic nervous system responsible for rest-and-digest responses.
pre-Bötzinger complex: a brainstem region crucial for respiratory rhythm generation.
Tidal volume: The air inhaled or exhaled during a normal breath.
vagal nerve activity: the function of the vagus nerve is to promote parasympathetic responses like reduced heart rate and stress.
References
Besedovsky, L., Lange, T., & Born, J. (2012). Sleep and immune function. Pflugers Archiv: European Journal of Physiology, 463(1), 121–137. https://doi.org/10.1007/s00424-011-1044-0
Boudewyns, A., Van de Heyning, P., De Backer, W., & De Cock, W. (2000). Pathophysiology and treatment of sleep-disordered breathing. Current Opinion in Pulmonary Medicine, 6(6), 471–476. https://doi.org/10.1097/00063198-200011000-00010
Cherniack, N. S., Longobardo, G. S., & Sant’Ambrogio, G. (2017). Control of alveolar recruitment during sighs. Respiratory Physiology & Neurobiology, 242, 23–29. https://doi.org/10.1016/j.resp.2017.03.004
Collins, G. T., & Eguibar, J. R. (2010). Neuropharmacology of yawning. Frontiers in Neurology, 1, 128. https://doi.org/10.3389/fneur.2010.00128
Gallup, A. C., & Gallup, G. G. Jr. (2007). Yawning as a brain cooling mechanism: Nasal breathing and the pharynx. Medical Hypotheses, 67(1), 6–14. https://doi.org/10.1016/j.mehy.2006.01.047
Harris, M., Glozier, N., & Ratnavadivel, R. (2009). Mood disorders and obstructive sleep apnea. Journal of Clinical Psychiatry, 70(9), 1182–1190. https://doi.org/10.4088/JCP.08m04878
Ju, Y. S., Lucey, B. P., & Holtzman, D. M. (2019). Sleep and Alzheimer’s disease pathology. Nature Reviews Neurology, 10(3), 115–122. https://doi.org/10.1038/s41582-019-0072-5
Komeili, G., Salamzadeh, J., & Komeili, F. (2020). Sighing and cardiovascular modulation. Acta Cardiologica, 75(1), 88–95. https://doi.org/10.1080/00015385.2019.1609404
Li, P., Janczewski, W. A., & Feldman, J. L. (2016). The sighing neural circuit in the brainstem. Nature, 530(7590), 293–297. https://doi.org/10.1038/nature16964
Meen, E., Park, K. J., & Song, H. J. (2021). Effects of mouth taping on snoring and nasal airflow. Journal of Sleep Medicine, 20(4), 183–190. https://doi.org/10.1016/j.sleep.2021.03.001
Platek, S. M., Critton, S. R., Myers, T. E., & Gallup, G. G. Jr. (2005). Contagious yawning and empathy. Cognitive Brain Research, 23(3), 448–452. https://doi.org/10.1016/j.cogbrainres.2005.06.009
Randerath, W., Verbraecken, J., & Andreas, S. (2018). Upper airway management during sleep: Position papers. European Respiratory Review, 27(150), 180033. https://doi.org/10.1183/16000617.0033-2018
Redline, S., Yenokyan, G., Gottlieb, D. J., & Shahar, E. (2010). Sleep-disordered breathing and cardiovascular events. New England Journal of Medicine, 363(13), 1205–1215. https://doi.org/10.1056/NEJMoa1000953
Sanna, A., Bovetto, S., & Cantalupi, L. (2012). Yawning neurobiology: PVN role in oxytocinergic activity. Neuropsychopharmacology, 37(4), 966–975. https://doi.org/10.1038/npp.2011.271
Somers, V. K., White, D. P., Amin, R., Abraham, W. T., Costa, F., & Thomas, R. J. (2008). Sleep apnea and cardiovascular disease. Journal of the American College of Cardiology, 52(8), 686–717. https://doi.org/10.1016/j.jacc.2008.05.002
Stokes, J. E., Patel, S. R., & Shepard, J. W. (2019). Mouth taping during CPAP therapy: Benefits and risks. Journal of Clinical Sleep Medicine, 15(6), 857–865. https://doi.org/10.5664/jcsm.7798
Sullivan, C. E., Issa, F. G., Berthon-Jones, M., & Eves, L. (1981). CPAP: A new treatment for obstructive sleep apnea. Lancet, 317(8225), 862–865. https://doi.org/10.1016/S0140-6736(81)92138-0
Tregear, S., Reston, J., Schoelles, K., & Phillips, B. (2009). Obstructive sleep apnea and motor vehicle crashes. Journal of Clinical Sleep Medicine, 5(6), 573–581. https://doi.org/10.5664/jcsm.27629
Vlemincx, E., Van Diest, I., & Van den Bergh, O. (2013). Sighing: A reset for stress and emotions. Biological Psychology, 93(2), 150–157. https://doi.org/10.1016/j.biopsycho.2012.11.012
Support Our Friends

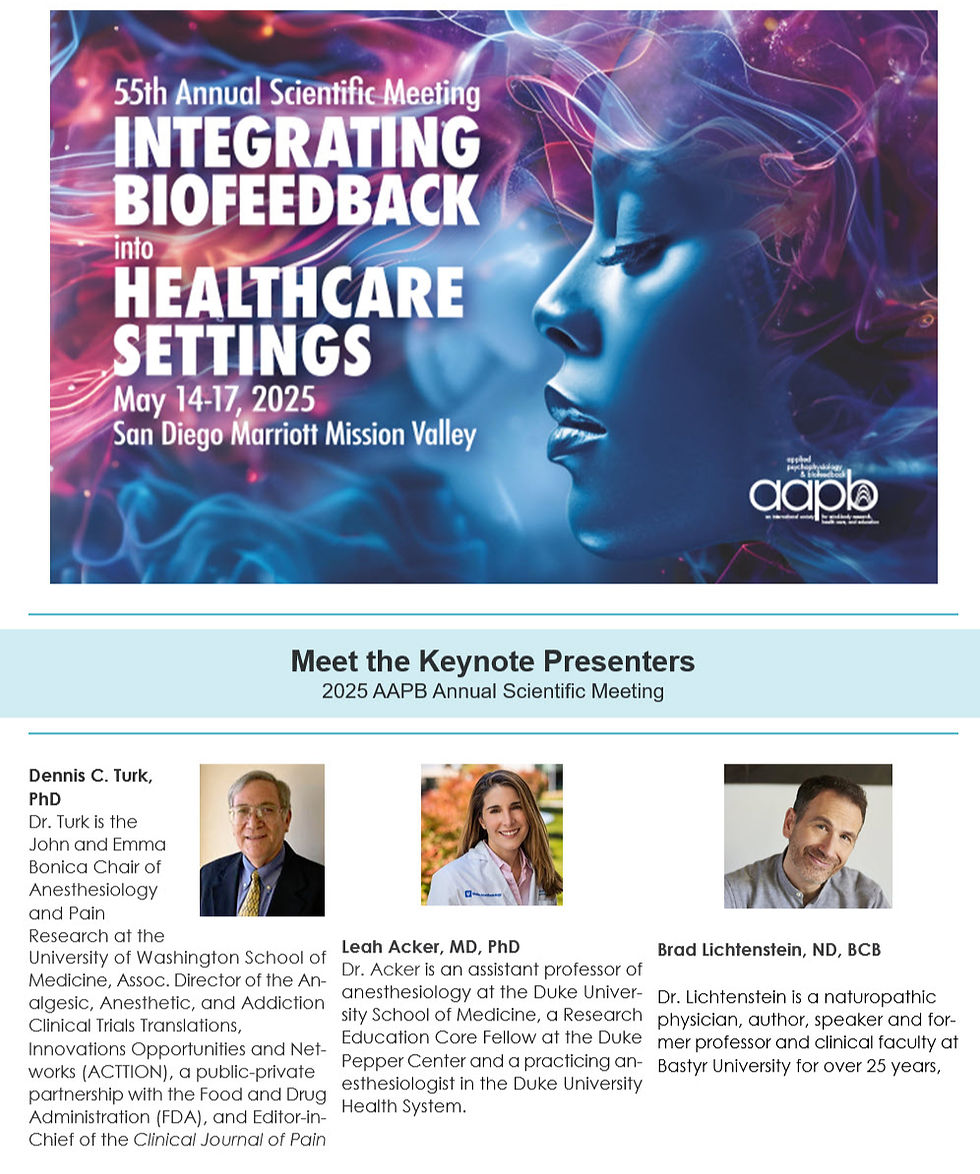
Comments