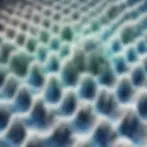
Gamma ranges from 30-100 Hz and includes the Sheer rhythm, which extends from 38-42 Hz (Buzsáki & Wang, 2012; Fries, 2015; Jensen et al., 2007). The difference in reported gamma frequency bands may reflect methodological differences and biological variability.
Methodological Differences
Different EEG systems and setups can have varying sensitivities and resolutions, affecting the frequency range they can accurately capture. High-density EEG systems may detect higher frequencies more reliably than standard setups (Hari & Puce, 2017; Nunez & Srinivasan, 2006).
Data processing variations, such as filters, window lengths, and analytical algorithms, can influence the detection and categorization of gamma frequencies. High-frequency oscillations can be more susceptible to noise and artifacts, such as muscle activity (EMG) or electrical interference. Different studies may apply varying degrees of filtering and artifact rejection, affecting the upper limit of detectable gamma frequencies (Niedermeyer & da Silva, 2004).
For these reasons, different studies might use different criteria for identifying gamma oscillations.
Biological Variability
Due to genetic, developmental, and health-related factors, the EEG patterns of different individuals can be significantly variable. This can lead to different observed upper limits for gamma oscillations (Başar, 2012; Ulhaas & Singer, 2010).
The cognitive or sensory task during EEG recording can affect the frequency and power of gamma oscillations. Different tasks may induce gamma activity in different frequency ranges.
Gamma oscillations can vary across different regions of the brain. For instance, the gamma frequency range observed in the visual cortex during visual processing tasks may differ from that observed in the hippocampus during memory tasks.
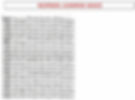
The overlap between gamma (30-100 Hz) and surface EMG (SEMG) (13-200 Hz) requires that clinicians examine the raw EEG to ensure that training does not reward muscle contraction. The movie below is a 19-channel BioTrace+ /NeXus-32 display of gamma activity © John S. Anderson. Brighter colors represent higher gamma amplitudes. Frequency histograms are displayed for each channel. Notice that the mean frequency falls between 38 and 39 Hz.
This post delves into the generators of gamma activity, its meaning in the context of brain function, and recent findings in the field. Research has linked gamma activity to cortical activation, cognitive processes, attention, emotion, and motor functions, with implications for conditions like ADHD, GAD, and epilepsy.
Gamma Rhythm Generators
Gamma oscillations are primarily generated by the synchronized activity of excitatory and inhibitory neurons in the brain, particularly within the cortical regions. The interplay between pyramidal neurons and interneurons, especially those expressing parvalbumin, is crucial for generating these high-frequency oscillations. These interneurons provide rhythmic inhibitory input to pyramidal cells, creating a network capable of producing gamma waves (Buzsáki & Wang, 2012).
Thalamocortical interactions also play a role in gamma generation. The thalamus, acting as a relay station for sensory information, can influence cortical gamma activity through its connections with the cortex. This interaction is particularly evident in sensory processing areas, where gamma oscillations are modulated by sensory input (Fries, 2015). Thalamocortical graphic © Netter.
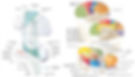
Furthermore, gamma activity is not uniformly distributed across the brain. Still, it is particularly prominent in regions involved in higher-order cognitive functions, such as the prefrontal cortex, hippocampus, and visual cortex. These areas utilize gamma oscillations for attention, memory encoding, and sensory perception (Bosman et al., 2014).
The Meaning of Gamma EEG Activity
Gamma oscillations have been implicated in various cognitive, perceptual, and motor functions, emotions, epilepsy, schizophrenia, Alzheimer's disease, and ADHD.
Binding Sensory Information
One of the primary roles of gamma activity is in binding sensory information. This concept, known as the binding problem, refers to how the brain integrates information from different sensory modalities to create a coherent perceptual experience. Gamma synchrony is thought to facilitate this process by providing a temporal framework that binds disparate neural signals (Singer, 2013).
Attention
In the context of attention, gamma oscillations have been shown to enhance the processing of relevant stimuli while suppressing irrelevant information. This selective attention mechanism is believed to be mediated by synchronizing gamma activity between different brain regions, thereby enhancing communication and processing efficiency (Jensen et al., 2007). Enhanced gamma activity is associated with better performance on cognitive tasks (Hasib et al., 2023).
Working and Long-Term Memory
Gamma activity is crucial for working memory and long-term memory encoding. Studies have demonstrated that gamma oscillations in the hippocampus and prefrontal cortex are involved in maintaining and manipulating information in working memory and encoding and retrieving long-term memories (Colgin, 2016).
Motor Performance
Gamma event-related synchronization (ERS) is linked to motor performance, with distinct low (35-50 Hz) and high (75-100 Hz) gamma bands showing different temporal and spatial characteristics during motor tasks (Amo et al., 2016, 2017; Crone et al., 1998). Enhanced gamma activity is observed during voluntary movements (Amo et al., 2016).
The Sheer rhythm is also associated with peak performance (Spydell, Ford, & Sheer, 1979). For example, 40-Hz activity is generated when athletes correct their balance after leaning forward on a balance board. Athletes who have sustained a concussion and suffer impaired balance do not increase 40-Hz power (Demos, 2019; Thompson & Thompson, 2015).
Emotions
Gamma fluctuations are associated with emotional experiences, with higher gamma activity linked to difficult emotions during worry inductions in GAD patients (Oathes, 2008).
Epilepsy
Increased gamma activity is noted in patients with primary generalized epilepsy, potentially serving as a marker for underlying dysfunctions and a prerequisite for seizures (Willoughby et al., 2003).
Schizophrenia
In schizophrenia, for example, deficits in gamma oscillations are thought to contribute to the cognitive impairments and sensory processing anomalies observed in patients (Uhlhaas & Singer, 2010).
Alzheimer's Disease
Recent studies have suggested that gamma oscillations might play a role in clearing amyloid-beta plaques, a hallmark of the disease. Optogenetic stimulation of gamma oscillations in mouse models has reduced amyloid-beta levels, pointing to potential therapeutic avenues (Iaccarino et al., 2016).
ADHD
Reduced gamma activity is observed in adults with ADHD, particularly in the right centroparietal region, correlating with symptom severity and suggesting altered network function (Tombor et al., 2019).
Recent Findings
Advancements in neuroimaging and electrophysiological techniques have allowed for more precise mapping of gamma activity and its sources. Magnetoencephalography (MEG) and high-density EEG have provided more detailed spatial and temporal resolution, enhancing our understanding of gamma oscillations in health and disease (Hari & Puce, 2017).
Conclusion
Gamma oscillations in EEG, ranging from 30-100 Hz, are critical for numerous brain functions, including cognitive processes, attention, memory, motor functions, and emotional experiences. This range includes the Sheer rhythm (38-42 Hz). Variations in reported gamma frequency bands stem from differences in EEG methodologies, such as system sensitivity and data processing techniques, and biological variability among individuals. High-density EEG systems can capture higher frequencies more reliably than standard setups. Gamma oscillations are generated by the synchronized activity of excitatory and inhibitory neurons, particularly in cortical regions, and their interplay is essential for producing these high-frequency waves.
Gamma oscillations play a vital role in binding sensory information, a process known as the binding problem, which integrates information from different sensory modalities to create a coherent perceptual experience. In the context of attention, gamma oscillations enhance the processing of relevant stimuli while suppressing irrelevant information, thereby improving cognitive task performance. Gamma activity is crucial for both working memory and long-term memory encoding, particularly in the hippocampus and prefrontal cortex.
Motor performance is also linked to gamma oscillations, with distinct low (35-50 Hz) and high (75-100 Hz) gamma bands showing different characteristics during motor tasks. The Sheer rhythm is associated with peak performance, such as athletes correcting their balance, where increased 40-Hz activity is observed. Emotional experiences are also linked to gamma fluctuations, with higher gamma activity correlating with difficult emotions in conditions like generalized anxiety disorder (GAD).
Gamma oscillations are implicated in several neurological and psychiatric conditions. In epilepsy, increased gamma activity is observed and may serve as a marker for underlying dysfunctions. In schizophrenia, deficits in gamma oscillations contribute to cognitive impairments and sensory processing anomalies. Alzheimer's disease research suggests that gamma oscillations might help clear amyloid-beta plaques, pointing to potential therapeutic avenues. In ADHD, reduced gamma activity is noted, particularly in the right centroparietal region, correlating with symptom severity.
Recent advancements in neuroimaging, such as magnetoencephalography (MEG) and high-density EEG, have improved the spatial and temporal resolution of gamma activity mapping, enhancing the understanding of gamma oscillations in both healthy and diseased states. These technologies allow for more precise identification of gamma rhythm sources and their roles in brain function.
Gamma oscillations' importance in various brain functions and their implications in several conditions highlight the need for continued research to fully elucidate their mechanisms and therapeutic potential.
Glossary
amyloid-beta: a protein fragment that accumulates in the brains of individuals with Alzheimer's disease, forming plaques.
attention: the cognitive process of selectively concentrating on one aspect of the environment while ignoring others.
binding problem: the question of how the brain integrates information from different sensory modalities to create a coherent perceptual experience.
cognitive processes: mental activities involved in acquiring, storing, and using knowledge.
electrophysiological techniques: methods used to study the electrical properties of biological cells and tissues.
excitatory neurons: neurons that increase the likelihood of a firing action potential in the recipient neuron.
gamma event-related synchronization (ERS): a phenomenon where gamma oscillations (30-100 Hz) in the brain become more synchronized in response to a specific event or stimulus, often associated with increased neural processing and cognitive functions such as attention and memory encoding.
gamma oscillations: brain waves in the frequency range of approximately 30 to 100 Hz, associated with various cognitive functions.
hippocampus: a brain region involved in the formation and retrieval of memories.
inhibitory neurons: neurons that decrease the likelihood of a firing action potential in the recipient neuron.
long-term memory: the storage of information over an extended period.
magnetoencephalography (MEG): a neuroimaging technique for mapping brain activity by recording magnetic fields produced by electrical currents occurring naturally in the brain.
neural oscillations: rhythmic or repetitive neural activity in the central nervous system.
optogenetic stimulation: a technique that uses light to control neurons genetically modified to express light-sensitive ion channels. This method allows precise control over the activity of specific neurons in the brain, enabling researchers to study the functions of neural circuits and their roles in behavior and neurological conditions.
parvalbumin: a protein expressed in certain inhibitory interneurons.
prefrontal cortex: a part of the brain involved in complex cognitive behavior, decision-making, and moderating social behavior.
schizophrenia: a chronic brain disorder characterized by symptoms such as hallucinations, delusions, and cognitive impairments.
selective attention: the process by which a person can selectively pick out one message from a mixture of messages occurring simultaneously.
sensory perception: the process of recognizing and interpreting sensory stimuli.
Sheer rhythm: a brain oscillation characterized by high-frequency activity (38-42 Hz) that occurs in short, transient bursts. These bursts are typically synchronized across large neural populations and are thought to play a role in the timing and coordination of neuronal activity, particularly in relation to cognitive processes and sensory perception.
surface EMG (SEMG): a non-invasive technique used to measure and record the electrical activity produced by skeletal muscles. Sensors placed on the skin's surface detect the electrical potentials muscle fibers generate during contraction, providing information about muscle function, activation patterns, and fatigue.
synchrony: the simultaneous occurrence of events or processes, in this context, the coordinated activity of neurons.
temporal framework: the timing structure that allows for the coordination of neural activity.
thalamocortical interactions: the connections and communications between the thalamus and the cerebral cortex.
working memory: a cognitive system responsible for temporarily holding information available for processing.
References
Amo, C., Castillo, M., Barea, R., Santiago, L., Martínez-Arribas, A., Amo-López, P., & Boquete, L. (2016). Induced gamma-band activity during voluntary movement: EEG analysis for clinical purposes. Motor Control, 20(4), 409-28. https://doi.org/10.1123/mc.2015-0010 Amo, C., Santiago, L., Barea, R., López-Dorado, A., & Boquete, L. (2017). Analysis of Ggamma-band activity from human EEG using empirical mode decomposition. Sensors (Basel, Switzerland), 17. https://doi.org/10.3390/s17050989 Bosman, C. A., Lansink, C. S., & Pennartz, C. M. (2014). Functions of gamma-band synchronization in cognition: From single circuits to functional diversity across cortical and subcortical systems. European Journal of Neuroscience, 39(11), 1982-1999. https://doi.org/10.1111/ejn.12606
Buzsáki, G., & Wang, X. J. (2012). Mechanisms of gamma oscillations. Annual Review of Neuroscience, 35, 203–225. https://doi.org/10.1146/annurev-neuro-062111-150444
Colgin, L. L. (2016). Rhythms of the hippocampal network. Nature Reviews Neuroscience, 17(4), 239-249. Crone, N., Miglioretti, D., Gordon, B., & Lesser, R. (1998). Functional mapping of human sensorimotor cortex with electrocorticographic spectral analysis. II. Event-related synchronization in the gamma band. Brain: A Journal of Neurology, 121( Pt 12), 2301-15. https://doi.org/10.1093/BRAIN/121.12.2301 Demos, J. N. (2019). Getting started with neurofeedback (2nd ed.). W. W. Norton & Company. Fries P. (2015). Rhythms for Cognition: Communication through coherence. Neuron, 88(1), 220–235. https://doi.org/10.1016/j.neuron.2015.09.034
Hari, R., & Puce, A. (2017). MEG-EEG primer. Oxford University Press.
Hasib, T., & Vengadasalam, V. (2023). Analysing gamma frequency components in EEG signals: A comprehensive extraction approach. Journal of Informatics and Web Engineering. https://doi.org/10.33093/jiwe.2023.2.2.11 Iaccarino, H. F., Singer, A. C., Martorell, A. J., Rudenko, A., Gao, F., Gillingham, T. Z., Mathys, H., Seo, J., Kritskiy, O., Abdurrob, F., Adaikkan, C., Canter, R. G., Rueda, R., Brown, E. N., Boyden, E. S., & Tsai, L. H. (2016). Gamma frequency entrainment attenuates amyloid load and modifies microglia. Nature, 540(7632), 230–235. https://doi.org/10.1038/nature20587
Jensen, O., Kaiser, J., & Lachaux, J. P. (2007). Human gamma-frequency oscillations associated with attention and memory. Trends in Neurosciences, 30(7), 317–324. https://doi.org/10.1016/j.tins.2007.05.001
Niedermeyer, E., & da Silva, F. L. (2004). Electroencephalography: Basic principles, clinical applications, and related fields. Lippincott Williams & Wilkins.
Oathes, D., Ray, W., Yamasaki, A., Borkovec, T., Castonguay, L., Newman, M., & Nitschke, J. (2008). Worry, generalized anxiety disorder, and emotion: Evidence from the EEG gamma band. Biological Psychology, 79, 165-170. https://doi.org/10.1016/j.biopsycho.2008.04.005
Singer W. (2013). Cortical dynamics revisited. Trends in Cognitive Sciences, 17(12), 616–626. https://doi.org/10.1016/j.tics.2013.09.006
Spydell, J. D., Ford, M. R., & Sheer, D. E. (1979). Task dependent cerebral lateralization of the 40 Hertz EEG rhythm. Psychophysiology, 16(4), 347–350. https://doi.org/10.1111/j.1469-8986.1979.tb01474.x
Thompson, M., & Thompson, L. (2015). The neurofeedback book: An introduction to basic concepts in applied psychophysiology (2nd ed.). Association for Applied Psychophysiology and Biofeedback.
Tombor, L., Kakuszi, B., Papp, S., Réthelyi, J., Bitter, I., & Czobor, P. (2019). Decreased resting gamma activity in adult attention deficit/hyperactivity disorder. The World Journal of Biological Psychiatry, 20, 691 - 702. https://doi.org/10.1080/15622975.2018.1441547
Uhlhaas, P. J., & Singer, W. (2010). Abnormal neural oscillations and synchrony in schizophrenia. Nature Reviews. Neuroscience, 11(2), 100–113. https://doi.org/10.1038/nrn2774
Willoughby, J., Fitzgibbon, S., Pope, K., Mackenzie, L., Medvedev, A., Clark, C., Davey, M., & Wilcox, R. (2003). Persistent abnormality detected in the non-ictal electroencephalogram in primary generalised epilepsy. Journal of Neurology, Neurosurgery & Psychiatry, 74, 51 - 55. https://doi.org/10.1136/jnnp.74.1.51.
Learn MoreLearn More
Support Our Friends at ISNR and AAPB

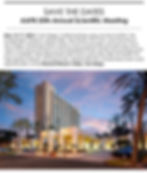