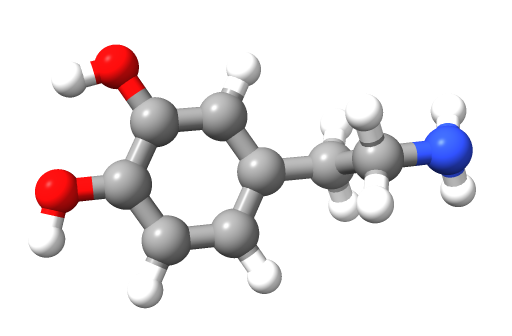
Dopamine is a neurotransmitter that regulates movement, cognition, reward, salience, and motivation.
It belongs to the catecholamine family, which also includes norepinephrine and epinephrine, all derived from the amino acid tyrosine. Dopamine's synthesis begins with the conversion of tyrosine into L-DOPA by the enzyme tyrosine hydroxylase. L-DOPA is then converted into dopamine by aromatic L-amino acid decarboxylase (Carlsson, 1959). As a precursor for norepinephrine and epinephrine, dopamine serves multiple functions in the central and peripheral nervous systems.
Dopamine Research from the 1950s to the Present
The story of dopamine began in the mid-20th century when Swedish scientist Arvid Carlsson discovered that dopamine was not merely a precursor to norepinephrine, as previously thought, but a neurotransmitter in its own right. In the 1950s, Carlsson demonstrated that dopamine plays a crucial role in motor function, particularly in the basal ganglia, a brain region involved in movement control.
His research showed that dopamine depletion in the striatum led to animal motor deficits, which could be reversed by administering L-DOPA, a dopamine precursor (Carlsson, 1959). This finding was foundational in understanding the neurotransmitter’s role in Parkinson’s disease, a neurodegenerative disorder characterized by the death of dopamine-producing neurons in the substantia nigra. Carlsson’s work earned him the 2000 Nobel Prize and laid the groundwork for dopamine’s recognition as a key player in movement regulation. Basal ganglia graphic redrawn by minaanandag.
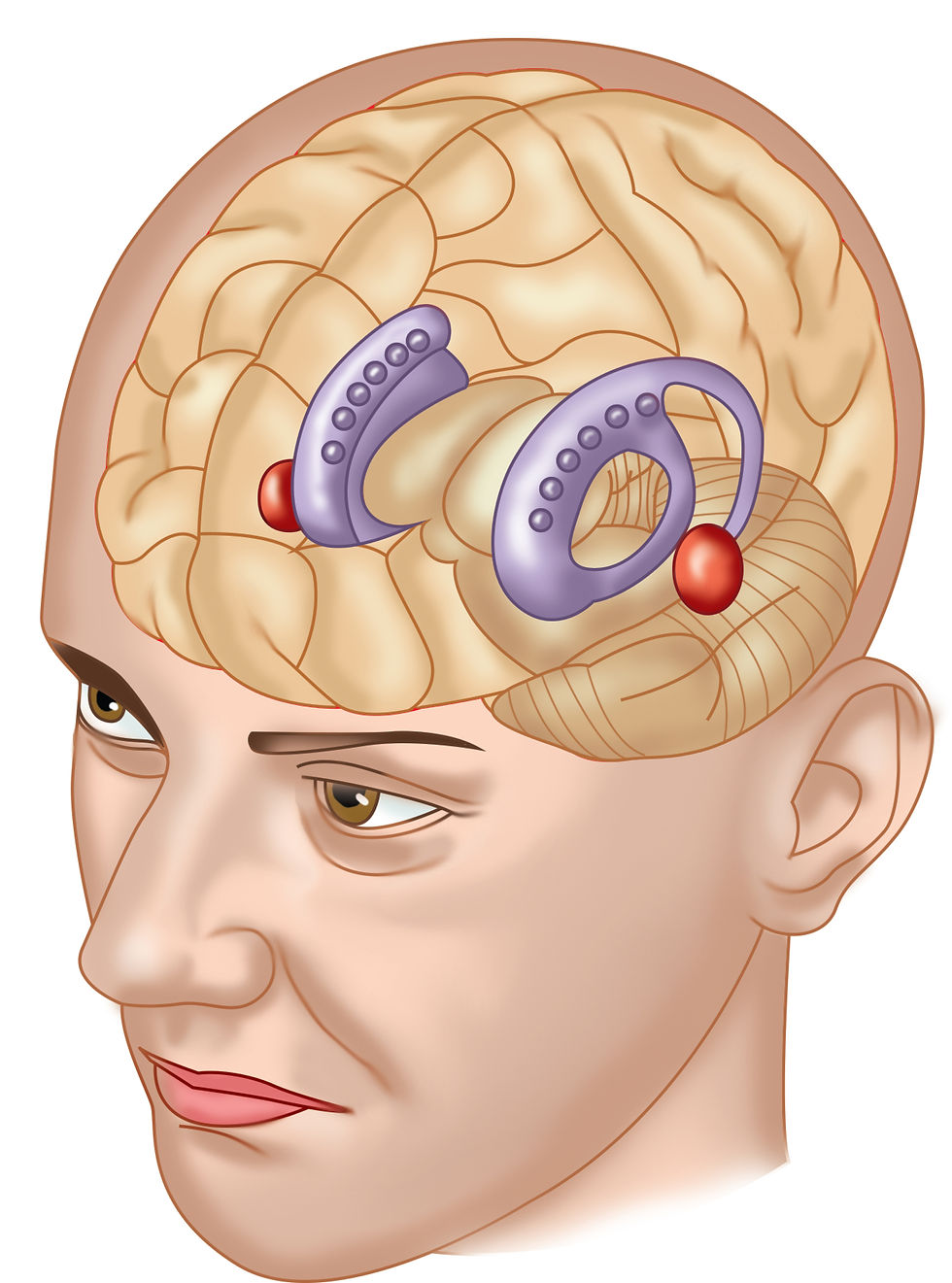
In 1959, Åke Bertler and Evald Rosengren reported that most of the brain's dopamine was located in the striatum, a region involved in motor control (Carlson, 2001).
1960s
The 1960s marked another major milestone when researchers Hornykiewicz and Ehringer linked dopamine depletion to human Parkinson’s disease (Hornykiewicz, 1966). Their post-mortem studies showed that patients with Parkinson’s had significantly reduced dopamine levels in the striatum, a region critical for motor function. This discovery led to the development of L-DOPA as a treatment for Parkinson’s disease, which remains one of the most effective therapies for managing the motor symptoms of the disorder (Lees et al., 2009). The development of high-dose oral DOPA treatment by George Cotzias in 1967 marked a significant breakthrough in PD therapy, which remains a cornerstone of treatment today (Björklund & Dunnett, 2007).
1970s and 1980s
In the 1970s and 1980s, dopamine’s role in reward and reinforcement learning became a major research focus. Early studies demonstrated that electrical stimulation of brain regions rich in dopamine, such as the ventral tegmental area (VTA), led to reinforcing effects in animals, causing them to repeatedly press a lever to receive the stimulation (Wise, 1980). This established dopamine as a key neurotransmitter in the brain’s reward system. Dopamine system graphic © Vasilisa Tsoy/Shutterstock.com.

Further research by Wolfram Schultz and colleagues in the 1980s provided more insight into how dopamine encodes rewards. Schultz discovered that dopamine neurons increased their firing rates in response to unexpected rewards. As animals learned to associate cues with rewards, the neurons responded to the predictive cues rather than the rewards themselves (Schultz et al., 1997).
This observation led to the concept of reward prediction error—the idea that dopamine signals the difference between expected and actual outcomes. Positive prediction errors occur when a reward is better than expected, while negative prediction errors arise when a reward is worse than expected. This framework revolutionized the understanding of how dopamine contributes to learning and decision-making.
1990s
The 1990s brought a deeper understanding of dopamine receptor subtypes and their distinct roles in brain function. Five dopamine receptor subtypes (D1-D5) were identified, with D1-like receptors (D1 and D5) primarily involved in stimulating intracellular signaling pathways and D2-like receptors (D2, D3, and D4) inhibiting these pathways (Missale et al., 1998). The discovery of these receptor subtypes allowed for more targeted pharmacological interventions, particularly in treating psychiatric disorders such as schizophrenia. Dopamine receptor graphic © Juan Gaertner/Shutterstock.com.
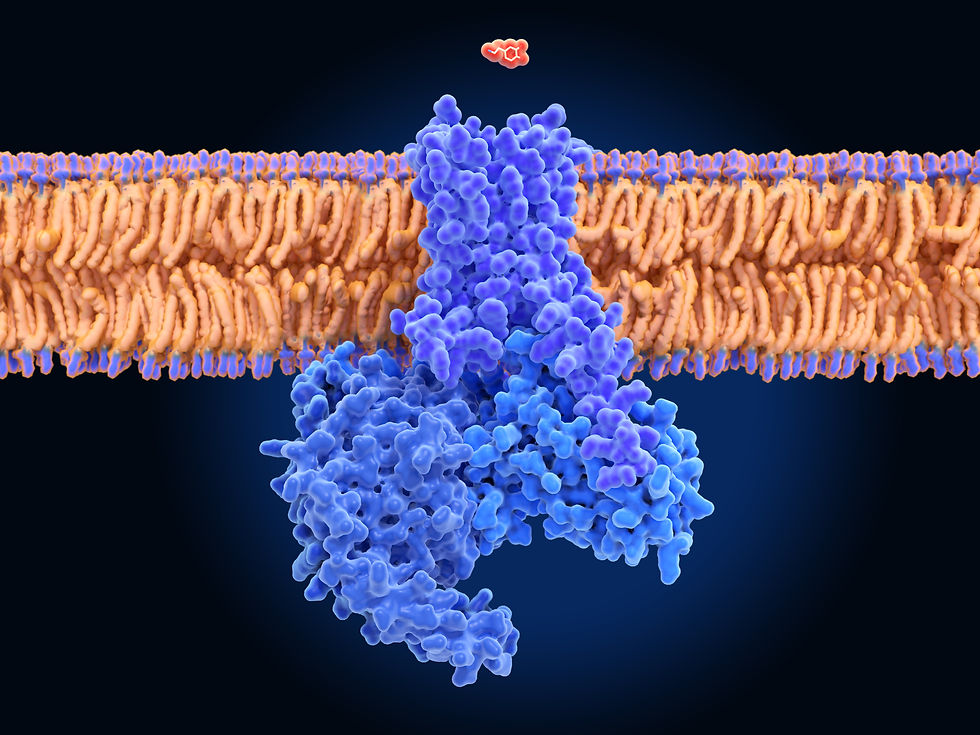
Caption: After binding extracellular dopamine (red), the dopamine receptor D1 (violet) activates a coupled G-protein (blue), starting an intracellular signal cascade.
The dopamine hypothesis of schizophrenia, which emerged in the 1970s and 1980s, posited that excessive dopamine activity in the mesolimbic pathway contributes to the positive symptoms of schizophrenia, such as hallucinations and delusions (Howes & Kapur, 2009). First-generation antipsychotic medications, which primarily act as D2 receptor antagonists, became the standard treatment for schizophrenia by reducing dopamine transmission in key brain areas. Chlorpromazine graphic © Sonis Photography/Shutterstock.com.
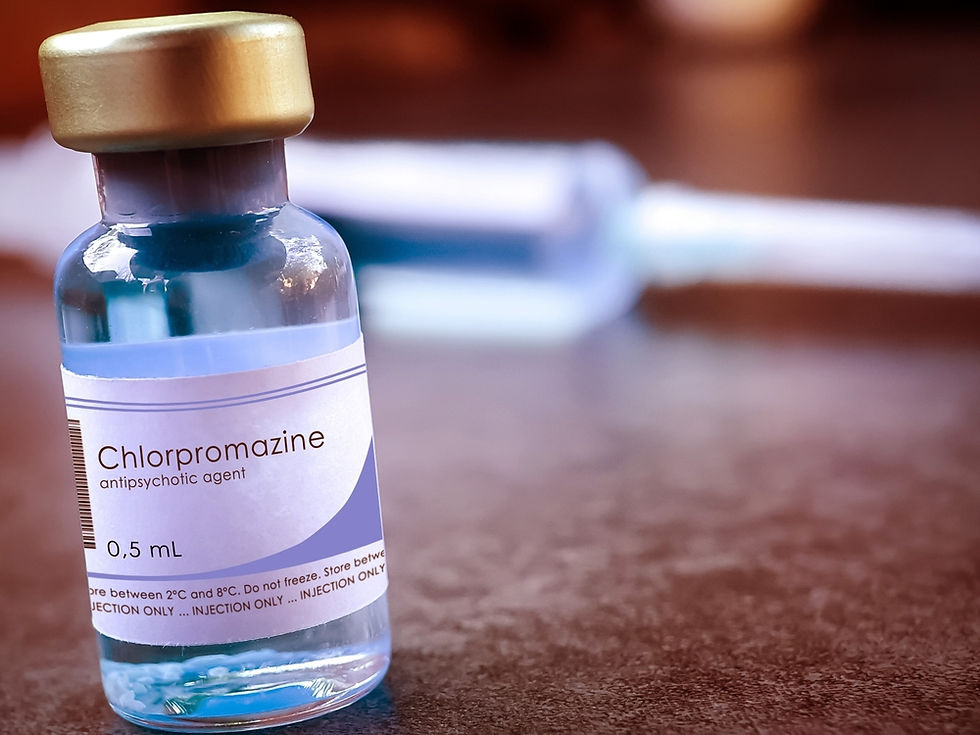
2000s
Dopamine’s role in cognitive and executive functions became clearer in the early 2000s. Researchers discovered that dopamine modulates motor and reward-related behaviors and influences higher-order cognitive processes such as working memory, attention, and decision-making, primarily through its action in the prefrontal cortex (Seamans & Yang, 2004). Dopaminergic modulation of the prefrontal cortex was found to be crucial for cognitive flexibility, goal-directed behavior, and the ability to adapt to changing environments. The cortex graphic © Vasilisa Tsoy/Shutterstock.com.

2010s
In recent years, advances in neuroimaging and optogenetics have further expanded the understanding of dopamine’s role in health and disease. Optogenetic techniques, which use light to control the activity of specific neurons, have allowed scientists to selectively activate or inhibit dopaminergic neurons, revealing their precise contributions to behaviors like reward-seeking, addiction, and decision-making (Tsai et al., 2009). Mouse optogenetic graphic retrieved from Wikipedia.
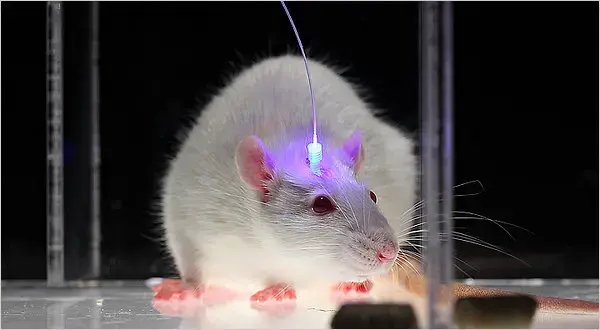
These techniques have also been used to investigate how dopamine contributes to psychiatric conditions such as depression and addiction, where dysregulated dopamine signaling plays a central role in altered reward processing and motivation (Treadway & Zald, 2011). In addiction, for instance, substances like cocaine and amphetamines increase dopamine release in the nucleus accumbens, leading to reinforcing effects that drive compulsive drug-seeking behaviors (Volkow & Morales, 2015).
Conclusion
Throughout its research history, dopamine has transitioned from being seen as merely a motor-related neurotransmitter to a critical modulator of cognition, motivation, reward, and emotion. The identification of its receptors, pathways, and complex roles in prediction and reward learning has not only advanced basic neuroscience but also led to effective treatments for conditions such as Parkinson’s disease, schizophrenia, and addiction. As research continues, newer techniques and models will likely shed even more light on the nuanced roles of dopamine in both normal and disordered brain function.
Google Illuminate Discussion
Please click on the podcast icon to hear a lively Google Illuminate discussion over this post.
Glossary
addiction: a chronic, relapsing disorder characterized by compulsive drug seeking, continued use despite harmful consequences, and long-lasting changes in the brain. It involves alterations in brain circuits related to reward, motivation, and control, with dopamine playing a central role in reinforcing drug-seeking behaviors.
aromatic L-amino acid decarboxylase: an enzyme that converts L-DOPA into dopamine.
catecholamine family: a group of neurotransmitters, including dopamine, norepinephrine, and epinephrine, involved in regulating various bodily functions.
D1-like receptors: a subtype of dopamine receptors that includes D1 and D5 receptors. These receptors are coupled to stimulatory G-proteins and increase the production of cyclic adenosine monophosphate (cAMP) when activated, enhancing excitatory signaling in the brain. They are involved in cognitive processes, motor control, and reward.
D2-like receptors: a subtype of dopamine receptors that includes D2, D3, and D4 receptors. These receptors are coupled to inhibitory G-proteins, reducing cAMP levels and decreasing neuronal excitability. D2-like receptors are involved in motor control, reward, and the regulation of various behavioral and cognitive functions.
D2-receptor antagonist: a type of drug that blocks or inhibits the action of dopamine at D2-like receptors, which are part of the dopamine receptor family. These receptors are found throughout the brain, particularly in regions involved in motor control, reward, and emotion. By binding to the D2 receptor, antagonists prevent dopamine from exerting its effects, thereby reducing dopaminergic signaling.
basal ganglia: a group of structures in the brain involved in movement regulation, reward processing, and habit formation.
dopamine hypothesis of schizophrenia: a theory that suggests the positive symptoms of schizophrenia, such as hallucinations and delusions, are caused by excessive dopamine activity in the mesolimbic pathway.
L-DOPA: a precursor to dopamine used to treat motor symptoms in Parkinson’s disease.
Mesolimbic pathway: A dopaminergic pathway involved in reward, motivation, and reinforcement learning.
norepinephrine: a neurotransmitter and hormone that is part of the catecholamine family. Norepinephrine is involved in arousal, alertness, attention, and the body’s fight-or-flight response. It also plays a role in regulating mood, stress, and cognitive functions, such as memory and learning.
nucleus accumbens: a brain region located in the basal forebrain that plays a central role in the brain's reward circuit. It is a major target of dopaminergic neurons from the ventral tegmental area and is involved in the processing of motivation, reward, pleasure, and reinforcement learning.
optogenetics: a technique that uses light to control the activity of specific neurons, allowing for precise investigation of brain circuits.
Parkinson's disorder: a neurodegenerative disorder characterized by the progressive loss of dopamine-producing neurons in the substantia nigra. This leads to motor symptoms such as tremors, rigidity, bradykinesia (slow movement), and postural instability. The disorder is also associated with cognitive and emotional changes.
prefrontal cortex: the front part of the brain responsible for executive functions such as decision-making, attention, and goal-directed behavior.
reward prediction error: a concept in which dopamine signals the difference between expected and actual rewards, guiding learning and decision-making.
salience: the importance or significance that the brain assigns to certain stimuli or experiences, making them stand out and capture attention. Dopamine plays a critical role in signaling salience by modulating neural activity in response to stimuli that are novel, rewarding, or relevant to survival. When a stimulus is considered salient, dopamine release in key brain regions like the nucleus accumbens and prefrontal cortex helps to prioritize the stimulus for further cognitive processing and behavioral response. striatum: a subcortical structure in the brain that plays a crucial role in various aspects of motor control, reward processing, and cognitive functions. It is part of the basal ganglia and is divided into the caudate nucleus and putamen.
tyrosine: an amino acid that serves as the precursor for dopamine synthesis.
tyrosine hydroxylase: an enzyme responsible for converting tyrosine into L-DOPA.
ventral tegmental area (VTA): a region in the midbrain where dopamine-producing neurons are located. The VTA is part of the brain’s reward system and projects to the nucleus accumbens, prefrontal cortex, and amygdala. It regulates reward, motivation, reinforcement learning, and the development of addictive behaviors.
References
Björklund, A., & Dunnett, S. (2007). Fifty years of dopamine research. Trends in Neurosciences, 30, 185-187. https://doi.org/10.1016/j.tins.2007.03.004
Carlsson, A. (1959). The occurrence, distribution, and physiological role of catecholamines in the brain. Pharmacological Reviews, 11(2), 490-493. https://doi.org/10.1124/pr.11.2.490
Carlsson, A. (2001). A paradigm shift in brain research. Science, 294(5544), 1021-1024. https://doi.org/10.1126/science.1066969
Hornykiewicz, O. (1966). Dopamine (3-hydroxytyramine) and brain function. Pharmacological Reviews, 18(2), 925-964. https://doi.org/10.1007/978-3-7091-2768-6_28
Howes, O. D., & Kapur, S. (2009). The dopamine hypothesis of schizophrenia: Version III—the final common pathway. Schizophrenia Bulletin, 35(3), 549-562. https://doi.org/10.1093/schbul/sbp006
Lees, A. J., Hardy, J., & Revesz, T. (2009). Parkinson's disease. The Lancet, 373(9680), 2055-2066. https://doi.org/10.1016/S0140-6736(09)60492-X
Missale, C., Nash, S. R., Robinson, S. W., Jaber, M., & Caron, M. G. (1998). Dopamine receptors: From structure to function. Physiological Reviews, 78(1), 189-225. https://doi.org/10.1152/physrev.1998.78.1.189
Schultz, W., Dayan, P., & Montague, P. R. (1997). A neural substrate of prediction and reward. Science, 275(5306), 1593-1599. https://doi.org/10.1126/science.275.5306.1593
Seamans, J. K., & Yang, C. R. (2004). The principal features and mechanisms of dopamine modulation in the prefrontal cortex. Progress in Neurobiology, 74(1), 1-58. https://doi.org/10.1016/j.pneurobio.2004.05.006
Tsai, H. C., Zhang, F., Adamantidis, A., Stuber, G. D., Bonci, A., de Lecea, L., & Deisseroth, K. (2009). Phasic firing in dopaminergic neurons is sufficient for behavioral conditioning. Science, 324(5930), 1080-1084. https://doi.org/10.1126/science.1168878
Treadway, M. T., & Zald, D. H. (2011). Reconsidering anhedonia in depression: Lessons from translational neuroscience. Neuroscience & Biobehavioral Reviews, 35(3), 537-555. https://doi.org/10.1016/j
Support Our Friends

Thank you!