Brodmann Area Involvement in Clinical Disorders
- BioSource Faculty
- Nov 18, 2024
- 48 min read
Updated: Nov 20, 2024
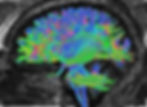
The opening graphic shows diffusion tensor imaging (DTI) used to trace the white matter tracts that connect different brain region. These tracts allow Brodmann areas to participate in brain networks.
The clinical correlations between various psychiatric and neurological disorders and specific Brodmann areas provide valuable insights into the underlying neural mechanisms of these conditions. Brodmann areas, defined by their distinct cytoarchitectonic characteristics, have been extensively studied in relation to numerous mental health and neurological disorders. Understanding the abnormalities in these regions can offer critical information for diagnosis, treatment, and the development of targeted therapies. This post explores the associations between specific Brodmann areas and disorders such as Alzheimer's disease, ADHD, autism spectrum disorder, bipolar disorder, frontotemporal dementia, major depressive disorder, panic disorder, PTSD, schizophrenia, and substance use disorder. Researchers have revised the Brodmann maps and correlated areas with their functions. The Brodmann maps below were contributed by Mark Dow, Research Assistant at the Brain Development Lab at the University of Oregon, to Wikimedia Commons.
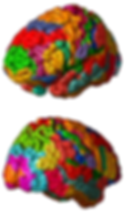
John Davis, PhD, BCN provided this Brodmann table for your reference.
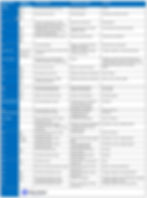
Alzheimer's Disease
Abnormalities in specific Brodmann areas are strongly associated with Alzheimer's disease. These include significant changes in areas 8, 10, 37, 19, 28, 39, 40, 9, 20, 25, 36, 38, and 47. These abnormalities manifest as hypoperfusion, neurofibrillary tangles, amyloid plaques, and synaptic changes, contributing to the cognitive deficits observed in AD patients. Understanding these changes can help in early diagnosis and targeted treatment strategies.

In Alzheimer's disease (AD), several Brodmann areas of the brain exhibit distinct pathologies and changes. Brodmann Area 37, located in the temporal gyrus, is characterized by significant hypoperfusion and neurofibrillary tangle (NFT) pathology, which impacts object naming and recognition memory (Thangavel et al., 2008, 2009). Similarly, Brodmann Area 19, part of the visual association cortex, shows dense NFTs, neuropil threads, and tau-immunoreactive neurites. These features are present in individuals with preclinical AD, mild cognitive impairment, or dementia, regardless of their cognitive status (Mckee et al., 2006).
The entorhinal cortex, represented by Brodmann Area 28, experiences substantial damage in AD, particularly with selective alterations in certain layers. This damage plays a crucial role in the memory deficits observed in the disease (Hosen, Hyman & Damasio, 1991). Brodmann Area 39, located in the angular gyrus, shows significant hypoperfusion in AD patients, which correlates with cognitive impairment (Transfaglia et al., 2009). Similarly, decreased perfusion in Brodmann Area 40, the supramarginal gyrus, distinguishes AD patients from those with mild cognitive impairment (Transfaglia et al., 2009). Delusional thoughts in Alzheimer's disease are associated with dysmetabolism in specific regions of the right prefrontal cortex: Brodmann's area 8, 10, and 47 (Sultzer et al., 2003).
In the frontal cortex, Brodmann Area 9 demonstrates synapse loss and changes in synaptic proteins, including increased PSD-95 expression. These alterations are closely associated with the severity of cognitive decline in AD (Leuba et al., 2008). Brodmann Area 20, in the inferior temporal cortex, exhibits hypoperfusion, which is linked to eating disorders in AD patients (Valotassiou et al., 2021).
Moreover, the subgenual area, represented by Brodmann Area 25, shows significant perfusion impairments in the early stages of AD, indicating its involvement in the disease's progression (Tranfaglia et al., 2009; Valotassiou et al., 2015, 2021). Finally, Brodmann Area 36, located in the parahippocampal gyrus, also experiences significant perfusion impairments in early AD, suggesting its crucial role during the initial stages of the disease (Tranfaglia et al., 2009; Valotassiou et al., 2015, 2021).
Attention-Deficit Hyperactivity Disorder (ADHD)
ADHD is associated with abnormalities in multiple Brodmann areas, including BAs 44/45 (Broca's area), 8/9, 10, 11, 46 (frontal regions), 7, 39, 40 (parietal regions), 4 (motor cortex), 30 (cingulate gyrus), 21, 38 (temporal regions), and 6 (premotor cortex). These abnormalities contribute to the diverse cognitive, attentional, and motor deficits observed in individuals with ADHD.

In individuals with ADHD, various brain regions show notable abnormalities. Broca's area, which includes Brodmann Areas 44 and 45, exhibits functional disturbances, particularly a lower fractional amplitude of low-frequency fluctuations (fALFF). This dysfunction is linked to language deficits, with different subtypes of ADHD affecting distinct parts of Broca's area: Area 44 in the inattentive subtype and Area 45 in the combined subtype (Pikusa & Jończyk, 2015; Silk et al, 2005).
In the frontal regions, children with ADHD display grey matter deficits in the right superior frontal gyrus (Brodmann Areas 8 and 9), suggesting disruptions in attentional networks (Overmeyer et al., 2001). Adolescents with ADHD show decreased activation in the middle frontal gyrus (Area 10) and the dorsolateral prefrontal cortex (Area 46), indicating widespread frontal lobe dysfunction (Silk et al., 2005). Furthermore, abnormalities in the ventromedial orbitofrontal cortex (Area 11) are linked to motivational deficits in adults with ADHD (Farré-Colomés et al., 2021).
The parietal regions, including the superior parietal lobe (Area 7) and the inferior parietal lobule (Areas 39 and 40), also show decreased activation in those with ADHD, implicating these areas in attentional and action-attentional systems (Silk et al., 2005). Additionally, children with ADHD exhibit decreased activation in the primary motor cortex (Area 4) during motor tasks, suggesting anomalies in motor development (Gaddis et al., 2015; Gilbert et al., 2011, 2019).
The right posterior cingulate gyrus (Area 30) shows grey matter deficits in children with ADHD, which may contribute to difficulties in attentional control (Overmeyer et al., 2001). Structural alterations are also observed in the temporal regions, with lower cortical thickness in the fusiform gyrus and temporal pole (Areas 21 and 38) in children with ADHD (Hoogman et al., 2019; Karalok et al., 2019; Lake et al., 2019; McLaughlin et al., 2014). Lastly, increased connectivity in the premotor cortex (Area 6) in individuals with ADHD may be associated with challenges in motor planning and execution (Hoshi & Tanji, 2007; Oldehinkel et al., 2016; Sörös et al., 2019; Suskauer et al., 2008).
Autism Spectrum Disorder (ASD)
Research indicates that abnormalities in Brodmann areas 24, 44, 45, and 10 are associated with Autism Spectrum Disorder. These abnormalities include changes in neuron size and density, gray matter volume, and functional connectivity, which are linked to the social and communication deficits characteristic of ASD. Further studies on these areas could enhance our understanding of the neural underpinnings of autism. In the anterior cingulate cortex (ACC), particularly in Brodmann Area 24, there is a notable reduction in cell size and cell packing density, specifically in layers 24b and 24c. Additionally, this region shows elevated levels of glial fibrillary acidic protein (GFAP) in the white matter, indicating increased astrocyte activation. During verbal memory tasks, a decrease in glucose metabolism is observed in BA24. Furthermore, there are dysregulated DNA methylation patterns in this area, impacting genes related to immune functions and synaptic membranes.

In the inferior frontal cortex, encompassing Brodmann Areas 44 and 45, there is a decrease in the size of pyramidal neurons, which play crucial roles in language processing and social behaviors (Jacot-Descombes et al., 2012). There is also reduced gray matter volume in the pars opercularis (BA44) and pars triangularis (BA45), which is linked to difficulties in social communication (Yamasaki et al., 2010).
The anterior prefrontal cortex, specifically Brodmann Area 10, exhibits abnormal functional connectivity between the right and left hemispheres. This abnormality correlates with the severity of social deficits (Kikuchi et al., 2013). Similar to BA24, BA10 also shows dysregulated DNA methylation, affecting genes involved in immune response and synaptic function (Nardone et al., 2014).
Other areas affected include the medial/cingulate regions (BAs 32, 24, and 25), where lower glucose metabolism is observed during verbal memory tasks (Deery et al., 2022; Hazlett et al., 2004, 2010). Additionally, there is a reduction in white matter volumes in the posterior frontal lobe and along the cingulate arch (Aalst et al., 2021; Gardener et al., 2016; .
Bipolar Disorder (BD)
Research indicates that abnormalities in several Brodmann areas, including BAs 9, 24, 38, 41, 42, 46, and 10, are associated with bipolar disorder. These abnormalities span structural changes, such as reduced glial numbers and cortical volume, as well as functional and molecular disruptions, including altered gene expression and DNA methylation. Understanding these abnormalities can provide insights into the pathophysiology of BD and aid in the development of targeted treatments. In Brodmann Area 9, part of the dorsolateral prefrontal cortex, there are abnormalities in DNA methylation and gene expression, indicating its role in the pathophysiology of bipolar disorder (BD). Additionally, this area shows decreased numbers and density of glial cells, suggesting potential disruptions in neurochemical regulation.

The subgenual prefrontal cortex, specifically Brodmann Area 24, exhibits reduced volume and fewer glial cells, which have been associated with familial forms of BD. This area is significant for mood regulation and reflects neurobiological changes linked to the disorder (Öngür et al., 1998; Scarr et al., 2019).
In the temporal pole, Brodmann Area 38 displays notable differences in DNA methylation, which are associated with BD, indicating its involvement in the molecular mechanisms underlying the disorder (Ho et al., 2019).
In the primary auditory cortex, comprising Brodmann Areas 41 and 42, decreased BOLD signals in response to auditory stimuli have been identified as potential biomarkers for BD, pointing to functional abnormalities in auditory processing (Okamoto et al., 2022).
Brodmann Area 46, another region of the dorsolateral prefrontal cortex, shows altered gene expression, with specific genes exhibiting differential expression in BD, suggesting disruptions in neuronal network functions (Nakatani et al., 2006; Visueta et al., 2012).
Finally, the prefrontal cortex, particularly Brodmann Area 10, also presents changes in gene expression in BD. These alterations highlight the complex regional-specific changes in cortical gene expression associated with the disorder (Scarr et al., 2019; Vizueta et al., 2012)
Frontotemporal Dementia (FTD)
Abnormalities in Brodmann areas 10R, 46R, 47R, 11, 32, 6, 8, 9, 38, 20, 21, 36L, and 37/19 are associated with frontotemporal dementia. These areas are primarily located in the frontal and temporal lobes and are linked to the behavioral and semantic deficits characteristic of FTD. Understanding these specific areas can aid in the accurate diagnosis and differentiation of FTD from other types of dementia. In frontotemporal dementia (FTD), there is notable hypoperfusion in the right anterior and dorsolateral prefrontal cortices, particularly in Brodmann Areas 10R and 46R, as well as in the orbital part of the right inferior frontal gyrus (BA 47R). This condition is also characterized by significant atrophy and reduced blood flow in the ventromedial frontal cortex, including Brodmann Areas 11 and 32, and the dorso-mesial frontal lobe, covering Areas 6, 8, and 9. These changes are closely associated with the behavioral symptoms observed in FTD.

The temporal lobe also shows significant involvement in FTD, with atrophy commonly seen in the left temporal pole (BA 38) and the inferolateral temporal lobe, particularly in Brodmann Areas 20 and 21 (Mummery et al., 1998, 2000). This pattern of atrophy is a hallmark of semantic dementia, a variant of FTD. Additionally, FTD patients exhibit hypoperfusion in the left parahippocampal gyrus (BA 36L) and the left posterior inferior temporal gyrus (BAs 37/19) (Valotassiou et al., 2021)
One of the distinguishing features of FTD compared to other dementias, such as mild cognitive impairment (MCI) and Alzheimer's disease (AD), is significant hypoperfusion in the frontal association cortex, specifically in Brodmann Area 47 (Palumbo et al., 2014; Tranfaglia et al., 2009). The involvement of specific regions, such as Areas 46R, 21L, and 25L/R, further aids in differentiating FTD from AD and other dementia subtypes (Valotassiou et al., 2012).
Major Depressive Disorder (MDD)
Research consistently implicates several Brodmann areas in the pathology of major depressive disorder. Key areas include the anterior cingulate cortex (BA 24 and BA 32), dorsolateral prefrontal cortex (BA 9 and BA 46), ventromedial prefrontal cortex (BA 10), orbitofrontal cortex (BA 13 and BA 47/12), and subgenual cingulate cortex (BA 25). These regions are associated with structural and functional abnormalities, altered connectivity, and changes in inflammatory markers, all contributing to the complex neurobiology of MDD. In the anterior cingulate cortex, particularly in Brodmann Areas 24 and 32, structural abnormalities and reduced volume in the subgenual part of BA 24 are linked to familial forms of major depressive disorder (MDD). Elevated levels of tumor necrosis factor (TNF) have been observed in BA 24 among MDD patients, suggesting the involvement of pro-inflammatory pathways. Furthermore, abnormalities in BA 32 have been associated with antidepressant treatment.

In the dorsolateral prefrontal cortex, including Brodmann Areas 9 and 46, both structural and functional abnormalities have been connected to MDD. In BA 9, changes in connectivity and increased fractional amplitude of low-frequency fluctuation (fALFF) are noted (Gao et al., 2021; Lai & Wu, 2015; Ye et al., 2012; Vasic et al. 2008; Zhukovsky et al., 2020). Additionally, elevated TNF levels in BA 46 indicate a role in the disorder's pathophysiology (Dean et al., 2010).
The ventromedial prefrontal cortex (BA 10) shows abnormal functional connectivity, which is linked to emotional regulation deficits in MDD patients (Almeida et al., 20111; Johnstone et al., 2007; Wackerhagen et al., 2017; Young et al., 2016). Epigenetic modifications, such as methylation changes, have also been identified in BA 9 and BA 38 (Ho et al., 2019).
In the orbitofrontal cortex, including Brodmann Areas 13 and 47/12, reduced functional connectivity in BA 13, a region involved in reward processing, is associated with depressive symptoms (Cheng et al., 2016). Conversely, increased functional connectivity in BA 47/12, related to non-reward and punishment, correlates with negative self-perception in MDD (Cheng et al., 2016).
The subgenual cingulate cortex (BA 25) exhibits reduced functional connectivity with regions involved in emotional regulation in MDD patients (Peng et al., 2020). Methylation changes in BA 25 have been consistently found in independent brain samples, highlighting its significance in the etiology of MDD (Åberg et al., 2018).
Panic Disorder
Research indicates that abnormalities in Brodmann areas 11, 25, 32, and 15, as well as regions within the prefrontal cortex and parahippocampal gyrus, are associated with panic disorder. These areas show altered activity and connectivity, contributing to the pathophysiology of PD. The findings highlight the involvement of the prefronto-limbic network and suggest that both hyperactivation and hypoactivation patterns in these regions play a role in the disorder. During panic attacks, there is a noticeable decrease in regional cerebral blood flow (rCBF) in the right orbitofrontal cortex (BA 11) and the prelimbic cortex (BA 25). This reduction is also observed in the anterior cingulate cortex (BA 32), where panic attacks lead to decreased rCBF. In the context of emotional processing in patients with panic disorder (PD), the anterior cingulate cortex (BA 32) exhibits mixed patterns of hyperactivation and hypoactivation.

The parahippocampal gyrus in PD patients shows significant abnormal asymmetry in cerebral blood flow. Similarly, the anterior temporal cortex (BA 15) experiences decreased rCBF during panic attacks (Hasler et al., 2007). Key regions within the prefronto-limbic network, particularly areas within the prefrontal cortex (PFC), show selective deficits in emotional processing among PD patients (Hasler et al., 2007; Shang et al., 2014). Additionally, dysfunctional communication within frontotemporal structures, including areas in the prefrontal cortex, is indicated by decreased coherence imaging values (Shang et al., 2014; Speer et al., 2003)
Increased resting-state functional connectivity between the thalamus and insula suggests excessive sensitivity to external information in PD patients. This heightened connectivity highlights a potential neural mechanism underlying the heightened responsiveness to stimuli often experienced by individuals with panic disorder (Feldker et al., 2016, 2019; Zhou et al., 2022).
Post-Traumatic Stress Disorder (PTSD)
PTSD is associated with abnormalities in several Brodmann areas, including the anterior cingulate cortex (BA 32 and BA 24), medial prefrontal cortex (BA 10 and BA 11), dorsolateral prefrontal cortex (BA 46), insula (BA 13), orbitofrontal cortex (BA 25), and sensorimotor areas (BA 4/6). These regions are involved in emotional regulation, memory processing, and response to trauma-related stimuli, highlighting the complex neural underpinnings of PTSD. In individuals with PTSD, the dorsolateral prefrontal cortex (DLPFC), specifically Brodmann area 46, shows decreased activity and signs of mitochondrial dysfunction. The anterior cingulate gyrus, located in Brodmann area 32, also exhibits less activation in PTSD subjects compared to those without PTSD. There are notable differences in functional connectivity in this region, suggesting its involvement in the recall of traumatic events.

The medial prefrontal cortex, encompassing Brodmann areas 10 and 11, shows reduced activation in PTSD subjects (Etkin & Wager, 2007; Herringa et al., 2012; Manthey et al., 2021; Sartory et al, 2013). The activation levels in BA 10, in particular, correlate with PTSD symptoms during threat processing. The insula, specifically Brodmann area 13, demonstrates increased delta slow waves, which are linked to worsening PTSD symptoms over time (Harricharan et al., 2019; Herringa et al., 2012; Rabinak et al., 2011). Additionally, differences in functional connectivity in the insula are observed between PTSD and non-PTSD individuals (Rabinak et al., 20111; Sripada et al., 2012; Zhang et al., 2016).
During symptom provocation in PTSD patients, there is increased regional cerebral blood flow (rCBF) in the right sensorimotor areas, specifically Brodmann areas 4 and 6 (Pissiota et al., 2000). Conversely, lowered rCBF is observed in the right retrosplenial cortex, which includes Brodmann areas 26, 29, and 30 (Pissiota et al., 2000).
Structural and functional changes in the hippocampus and amygdala are consistently observed in PTSD, highlighting their crucial roles in memory and emotional processing (Chen et al., 2018; Hull, 2002).
Schizophrenia
Research indicates that abnormalities in specific Brodmann areas, particularly in the frontal (BAs 4, 6, 8, 9, 10, 44, 46, 47), temporal (BAs 24, 25, 29, 30, 31), and cingulate (BAs 24, 25, 29, 30, 31) cortices, are associated with schizophrenia. These abnormalities include disrupted intercorrelations, altered receptor binding, gene expression changes, and structural deficits. The findings suggest that these regions play critical roles in the cognitive and functional impairments observed in schizophrenia, and they may serve as potential biomarkers for the disorder. Research has highlighted several key insights into the brain abnormalities associated with schizophrenia. Notably, significant reductions in gray matter volume have been observed in the left anterior hippocampus-amygdala, left parahippocampal gyrus, and left superior temporal gyrus. These reductions are particularly linked to thought disorders, with a correlation found between the volume of the left posterior superior temporal gyrus and the severity of these disorders.

Additionally, disruptions in D2 dopamine receptor patterns have been identified in specific regions of the temporal lobe, including the perirhinal, superior, and inferior temporal cortices (Goldsmith et al., 1997; Joyce et al., 1997). These areas correspond to Brodmann areas 20, 22, 37, 39, and 42. The observed disruptions are distinct to the temporal lobe and are not attributable to long-term antipsychotic treatment, suggesting a specific role in the manifestation of auditory hallucinations and other positive symptoms of schizophrenia.
In the context of schizotypal personality disorder, which shares some features with schizophrenia, lower fractional anisotropy (FA) has been observed in the left temporal lobe, particularly within Brodmann areas 20, 21, and 22 (Chan et al., 2018; Ellison-Wright & Bullmore, 2009; Hazlett et al., 2011; Lee et al., 2016). This finding indicates alterations in the white matter microstructure (Szeszko et al., 2008).
Moreover, schizophrenia is associated with a thinner cortex and reduced surface area in the temporal lobe, with the most significant reductions in cortical thickness observed at the temporal pole (Kaur et al., 2020; McCarley et al., 1999; Shenton et al., 1992) . There is also evidence of progressive volumetric changes over time, particularly a decrease in temporal white matter volume, indicating ongoing structural abnormalities in the brains of individuals with schizophrenia (Kaur et al., 2020; Mathew et al., 2014; Olabi et al., 2011).
Finally, enhanced functional connectivity between the left dorsolateral prefrontal cortex (DLPFC) and the left mid-posterior temporal lobe has been identified in patients experiencing their first episode of schizophrenia (Zhou et al., 2007). This altered connectivity pattern may play a role in the cognitive and perceptual disturbances characteristic of the disorder.
Substance Use Disorder (SUD)
Abnormalities in specific Brodmann areas, particularly BA 10, BA 24, BA 30, BA 18, BA 21/22, and BA 19, are associated with substance use disorder. These areas are involved in critical functions such as self-reflection, emotional regulation, memory, and executive function, which are often impaired in individuals with SUD.

In adolescents with substance use disorder (SUD), there is reduced activity in Brodmann Area 10, located in the superior, medial, and middle frontal gyrus (Dalwani et al., 2014). This reduction suggests difficulties in self-referential evaluation and future planning. The anterior cingulate cortex, specifically Brodmann Area 24, shows abnormal activity, impacting emotional behavior and executive function in individuals with SUD.
While studies have not found significant epigenome-wide differences in the dorsolateral prefrontal cortex (Brodmann Area 9), this area is still of interest in research on the molecular mechanisms underlying SUD. The retrosplenial cortex, particularly Brodmann Area 30, exhibits reduced activity, which is associated with impairments in memory and perspective-taking.
In the lingual gyrus (Brodmann Area 18), decreased activity is observed, potentially affecting visual processing and memory. The middle temporal gyrus, including Brodmann Areas 21 and 22, shows reduced activity, correlating with deficits in memory and foresight in patients with SUD. Interestingly, increased activity is noted in the cuneus (Brodmann Area 19) among SUD patients, although this observation is influenced by comorbid conditions such as depression and ADHD.
Conclusion
The examination of specific Brodmann areas reveals significant correlations with various psychiatric and neurological disorders, highlighting the importance of these brain regions in disease pathology. In Alzheimer's disease, cognitive deficits are linked to abnormalities in areas associated with memory and cognition. ADHD features disruptions in regions related to attention and executive function. Autism spectrum disorder is characterized by changes in areas affecting social and communication skills. Bipolar disorder involves alterations in areas associated with mood regulation.
Frontotemporal dementia primarily affects regions responsible for behavior and language, while major depressive disorder shows abnormalities in areas involved in emotion regulation. Panic disorder and PTSD both involve the prefronto-limbic network, impacting emotional and stress responses. Schizophrenia is associated with widespread disruptions in areas affecting cognition and perception, and substance use disorder involves regions critical for executive function and emotional regulation. Understanding these specific brain region abnormalities offers insights into the mechanisms underlying these disorders and aids in developing targeted treatments.
Glossary
Alzheimer's disease (AD): a progressive neurodegenerative disorder characterized by memory loss, cognitive decline, and behavioral changes. It is associated with the accumulation of amyloid plaques and neurofibrillary tangles, particularly in the hippocampus and cortex.
amyloid plaques: extracellular deposits of beta-amyloid protein found in the brains of individuals with Alzheimer's disease. These plaques are believed to disrupt cell-to-cell communication and trigger inflammatory responses. angular gyrus: located in the parietal lobe near the junction of the temporal and occipital lobes, the angular gyrus corresponds to Brodmann area 39. It plays a role in language processing, attention, spatial cognition, and integration of sensory information. anterior cingulate cortex (ACC): located in the medial portion of the frontal lobes, the anterior cingulate cortex encompasses Brodmann areas 24, 25, 32, and 33. It plays a role in executive function, emotional regulation, attention, conflict monitoring, and error detection.
anterior prefrontal cortex (aPFC): found in the most anterior region of the prefrontal cortex, the anterior prefrontal cortex includes Brodmann areas 10 and 11. It involves complex cognitive processes such as planning, decision-making, working memory, and abstract reasoning.
Attention-Deficit Hyperactivity Disorder (ADHD): a neurodevelopmental disorder characterized by inattention, hyperactivity, and impulsivity. It is associated with abnormalities in brain regions such as the prefrontal cortex and basal ganglia.
auditory cortex: situated in the superior temporal gyrus, the auditory cortex encompasses Brodmann areas 41 and 42. It is responsible for processing and interpreting auditory information.
autism spectrum disorder (ASD): a neurodevelopmental disorder characterized by deficits in social communication and interaction, along with restricted and repetitive behaviors. It involves atypical brain development and connectivity, particularly in areas such as the temporal lobes and frontal cortex.
bipolar disorder (BD): a mental health disorder characterized by extreme mood swings, including manic and depressive episodes. It is associated with abnormalities in brain regions involved in mood regulation, such as the prefrontal cortex and amygdala.
blood-oxygen-level-dependent (BOLD) signal: a measure used in functional magnetic resonance imaging (fMRI) to detect changes in blood flow and oxygenation levels in the brain, reflecting neural activity.
Brodmann areas: regions of the cerebral cortex defined based on their cytoarchitectonic characteristics, each area associated with specific functions. For example, Brodmann area 17 corresponds to the primary visual cortex.
cingulate arch: a neural structure comprising the cingulate gyrus, part of the limbic system, involved in emotion formation, processing, learning, and memory.
cingulate cortex: a part of the limbic system, the cingulate cortex is situated in the medial aspects of the frontal and parietal lobes, covering Brodmann areas 23, 24, 30, 31, and 33. It involves emotion processing, memory, attention, and cognitive control.
D2 dopamine receptor: a subtype of dopamine receptor implicated in the regulation of mood, motivation, and reward. Abnormalities in these receptors are associated with psychiatric disorders such as schizophrenia.
DNA methylation: an epigenetic mechanism involving the addition of a methyl group to DNA, affecting gene expression without altering the DNA sequence. It plays a role in development, cellular differentiation, and disease.
dorsal anterior cingulate cortex (dACC): located in the dorsal region of the anterior cingulate cortex, the dorsal anterior cingulate cortex includes Brodmann areas 24 and 32. It plays a role in cognitive control, decision-making, and conflict monitoring.
dorsal entorhinal cortex (DEC): situated in the medial temporal lobe, the dorsal entorhinal cortex comprises parts of Brodmann area 28. It is involved in spatial memory and navigation.
dorsolateral prefrontal cortex (DLPFC): found in the lateral region of the prefrontal cortex, the dorsolateral prefrontal cortex includes Brodmann areas 9, 46, and parts of 8 and 10. It involves working memory, cognitive flexibility, planning, and abstract reasoning.
dorsal posterior cingulate cortex (dPCC): located in the posterior part of the cingulate cortex, the dorsal posterior cingulate cortex covers Brodmann area 31. It is involved in self-referential thought, memory, and spatial awareness.
dorso-mesial frontal lobe: the medial part of the frontal lobe, involved in self-referential thought, moral decision-making, and social cognition.
dysmetabolism: abnormal metabolism that can affect brain function and is often associated with metabolic disorders such as diabetes.
ectosplenial cerebral cortex: part of the retrosplenial cortex, the ectosplenial cerebral cortex is situated within the cingulate cortex and covers Brodmann area 29. It plays a role in spatial memory, navigation, and contextual processing.
epigenetic modifications: changes in gene expression that do not involve alterations to the DNA sequence, often through mechanisms such as DNA methylation and histone modification.
executive functions: a set of cognitive processes, including working memory, flexible thinking, and self-control, that enable goal-directed behavior.
fractional amplitude of low-frequency fluctuations (fALFF): a measure used in resting-state fMRI to assess the amplitude of low-frequency oscillations in the brain, reflecting spontaneous neural activity.
fractional anisotropy (FA): a measure derived from diffusion tensor imaging (DTI) that quantifies the directional coherence of water diffusion in the brain, often used to assess white matter integrity.
frontal eye field (FEF): located in the anterior part of the middle frontal gyrus, the frontal eye field corresponds to Brodmann area 8. It is involved in voluntary eye movement control and visual attention.
frontal lobe dysfunction: impairment in the functions associated with the frontal lobes, including executive functions, emotional regulation, and social behavior.
frontotemporal dementia (FTD): a group of neurodegenerative disorders characterized by progressive damage to the frontal and temporal lobes, leading to changes in personality, behavior, and language.
frontotemporal lobar degeneration (FTLD): a group of rare, progressive neurodegenerative disorders characterized by atrophy and degeneration of the frontal and temporal lobes of the brain, leading to significant changes in personality, behavior, language, and motor function. FTLD subtypes include frontotemporal dementia (FTD), primary progressive aphasia (PPA), and semantic variant of primary progressive aphasia (SVPPA), among others. FTLD is often diagnosed in people under 65 and is distinct from Alzheimer's disease, although it can share similar symptoms.
functional connectivity: the temporal correlation between spatially remote neurophysiological events, often measured using fMRI to assess the synchrony between different brain regions.
fusiform gyrus: situated in the ventral region of the temporal and occipital lobes, the fusiform gyrus includes Brodmann areas 37 and parts of 19 and 20. It is involved in face recognition, object recognition, and color and visual form processing. glial fibrillary acidic protein (GFAP): an intermediate filament protein expressed by astrocytes in the central nervous system, often used as a marker for astrocytic activity and gliosis.
grey matter deficits: reductions in the volume or density of grey matter in the brain, often associated with neurodegenerative and psychiatric disorders.
hyperactivation: increased neural activity in response to stimuli, often observed in specific brain regions in various neurological and psychiatric conditions.
hypoactivation: reduced neural activity in response to stimuli, often observed in specific brain regions in various neurological and psychiatric conditions.
hypoperfusion: a state of reduced blood flow to a specific region of the brain, often detected in brain imaging studies such as functional magnetic resonance imaging (fMRI), positron emission tomography (PET), or single photon emission tomography (SPET). Hypoperfusion can indicate decreased neural activity, impaired cognitive function, or underlying neuropathology, and is often associated with various neurological and psychiatric conditions, such as Alzheimer's disease, frontotemporal dementia, stroke, and depression.
inferior frontal cortex: the lower part of the frontal lobe, involved in language production, social cognition, and motor function. It includes regions such as the pars opercularis and pars triangularis.
inferior temporal gyrus (ITG): located in the inferior temporal lobe region, the inferior temporal gyrus encompasses Brodmann areas 20 and 21. It plays a role in visual object recognition and semantic memory.
inferolateral temporal lobe: the lower and outer portion of the temporal lobe, involved in visual processing, particularly related to object recognition and memory.
insular cortex (insula): situated within the lateral sulcus, the insular cortex covers Brodmann areas 13, 14, 15, and 16. It is involved in processing emotions, interoception, self-awareness, pain perception, and taste sensation.
left mid-posterior temporal lobe: a region in the left hemisphere of the temporal lobe, involved in language processing and semantic memory.
Major Depressive Disorder (MDD): a psychological disorder characterized by persistent feelings of sadness, loss of interest, and other cognitive and physical symptoms. It is associated with abnormalities in brain regions such as the prefrontal cortex, amygdala, and hippocampus.
middle frontal gyrus: a region of the frontal lobe involved in cognitive functions such as working memory, attention, and executive function. It corresponds to parts of Brodmann areas 9 and 46.
middle temporal gyrus (MTG): located in the middle region of the temporal lobe, the middle temporal gyrus encompasses Brodmann areas 21 and 37. It plays a role in language processing, semantic memory, and visual motion processing.
mild cognitive impairment (MCI): a condition characterized by noticeable cognitive decline that is not severe enough to interfere significantly with daily life. MCI can be a precursor to Alzheimer's disease and other dementias.
neurofibrillary tangles: intracellular aggregates of hyperphosphorylated tau protein found in the brains of individuals with Alzheimer's disease. These tangles disrupt neuronal function and contribute to neurodegeneration.
orbitofrontal cortex (OFC): found in the ventral part of the frontal lobes, the orbitofrontal cortex includes Brodmann areas 10, 11, 12, 13, 14, and 47. It involves decision-making, reward processing, emotional regulation, and social cognition.
panic disorder (PD): an anxiety disorder characterized by recurrent, unexpected panic attacks and persistent concern about future attacks. It involves dysfunction in brain regions such as the amygdala and prefrontal cortex.
parahippocampal gyrus: a region of the brain located in the medial temporal lobe, surrounding the hippocampus, involved in memory encoding and retrieval.
pars opercularis: located in the inferior frontal gyrus, the pars opercularis corresponds to Brodmann area 44. It plays a role in language production and is part of Broca's area.
pars orbitalis: situated in the ventral part of the inferior frontal gyrus, the pars orbitalis covers Brodmann area 47. It is involved in language processing, social cognition, and emotional regulation.
pars triangularis: located in the anterior part of the inferior frontal gyrus, the pars triangularis corresponds to Brodmann area 45. It is involved in language processing and is part of Broca's area.
parainsular area: found adjacent to the insular cortex, the parainsular area comprises parts of Brodmann areas 13 and 52. It involves auditory and somatosensory integration and processing pain and temperature sensations.
perirhinal cortex: situated in the medial temporal lobe, the perirhinal cortex encompasses Brodmann areas 35 and 36. It plays a role in object recognition, associative memory, and contextual processing.
Post-Traumatic Stress Disorder (PTSD): a psychological condition triggered by experiencing or witnessing a traumatic event. It involves dysfunction in brain regions such as the amygdala, hippocampus, and prefrontal cortex. prefrontal cortex (PFC): the front part of the frontal lobes, involved in complex cognitive behaviors such as decision-making, social behavior, and executive functions.
prefronto-limbic network: a neural circuit involving the prefrontal cortex and limbic system, crucial for emotion regulation, decision-making, and social behavior.
prelimbic cortex: a region in the prefrontal cortex associated with executive functions, decision-making, and emotional regulation.
primary gustatory cortex: located within the insular cortex, the primary gustatory cortex corresponds to Brodmann area 43. It is responsible for processing taste information.
primary motor cortex (M1): situated in the precentral gyrus, the primary motor cortex corresponds to Brodmann area 4. It is responsible for voluntary movement control.
primary somatosensory cortex (S1): located in the postcentral gyrus, the primary somatosensory cortex covers Brodmann areas 1, 2, and 3. It is responsible for processing somatosensory information, including touch, pain, temperature, and proprioception.
primary visual cortex (V1): situated in the calcarine sulcus within the occipital lobe, the primary visual cortex corresponds to Brodmann area 17. It is responsible for processing basic visual information.
pyriform cortex: located in the ventral part of the temporal lobe, the pyriform cortex (also known as the primary olfactory cortex) includes parts of Brodmann areas 27, 28, and 34. It is responsible for processing olfactory information.
retrosplenial cingulate cortex: found in the posterior part of the cingulate cortex, the retrosplenial cingulate cortex covers Brodmann areas 29 and 30. It is involved in spatial memory, navigation, and contextual processing.
retrosubicular area: located in the medial temporal lobe, the retrosubicular area is part of the parahippocampal gyrus and corresponds to Brodmann area 27. It is involved in spatial navigation and memory.
right posterior cingulate gyrus: a region located in the cingulate cortex, involved in processes related to self-reflection, memory, and visual-spatial orientation.
schizophrenia: a chronic psychiatric disorder characterized by delusions, hallucinations, disorganized thinking, and impaired social functioning. It involves dysregulation in dopaminergic pathways and structural abnormalities in the brain.
schizotypal personality disorder: a psychological condition characterized by pervasive patterns of social and interpersonal deficits, cognitive or perceptual distortions, and eccentric behavior.
secondary visual cortex (V2): situated adjacent to the primary visual cortex in the occipital lobe, the secondary visual cortex corresponds to Brodmann area 18. It is involved in processing visual information, including recognition of shapes, colors, and spatial orientation.
semantic dementia: a subtype of frontotemporal dementia characterized by the progressive loss of the ability to understand and generate language and recognize objects or faces, typically associated with atrophy in the temporal lobes.
semantic language functions: the cognitive processes that enable us to understand and use language to convey meaning, including understanding word meanings, interpreting sentences and texts, making inferences, recognizing semantic relationships, using context to disambiguate, understanding figurative language, and generating meaningful language. these functions are essential for effective communication, problem-solving, and learning, and are supported by a network of brain regions. impairments in semantic language functions can be seen in various neurological and psychiatric conditions.
somatosensory association cortex (SAC): located in the parietal lobe, the somatosensory association cortex encompasses Brodmann areas 5 and 7. It is involved in the integration and interpretation of somatosensory information, such as touch, pain, temperature, and proprioception.
subgenual area: a region in the medial prefrontal cortex associated with mood regulation and implicated in mood disorders such as depression. It corresponds to Brodmann area 25.
subgenual ventromedial prefrontal cortex (vmPFC): situated in the ventral part of the medial prefrontal cortex, the subgenual ventromedial prefrontal cortex includes parts of Brodmann areas 25, 32, and 14. It is involved in emotional regulation, decision-making, and social cognition.
substance use disorder (SUD): a condition characterized by the harmful or hazardous use of psychoactive substances, including alcohol and illicit drugs. It involves dysregulation in reward pathways and prefrontal cortex functioning.
superior parietal lobe: the upper portion of the parietal lobe involved in spatial orientation, attention, and the integration of sensory information.
supplementary motor cortex (SMA): located in the medial part of the superior frontal gyrus, the supplementary motor cortex corresponds to Brodmann area 6. It is involved in planning and coordinating complex movements and motor learning.
supramarginal gyrus: situated in the parietal lobe, the supramarginal gyrus is part of the inferior parietal lobule and corresponds to Brodmann area 40. It is involved in language processing, attention, and spatial cognition.
superior temporal gyrus (STG): located in the superior region of the temporal lobe, the superior temporal gyrus encompasses Brodmann areas 22, 41, and 42. It plays a role in auditory processing, language comprehension, and social cognition.
temporal gyrus: any of the gyri located in the temporal lobe, involved in auditory processing, language comprehension, and memory.
temporopolar area: situated in the most anterior part of the temporal lobe, the temporopolar area corresponds to Brodmann area 38. It is involved in olfactory processing, social cognition, and semantic memory.
thalamus: a large, dual-lobed mass of grey matter buried under the cerebral cortex, involved in relaying sensory and motor signals to the cerebral cortex and regulating consciousness, sleep, and alertness.
tumor necrosis factor (TNF): a cytokine involved in systemic inflammation and part of the body's immune response. It plays a role in the pathophysiology of various neurological and psychiatric conditions.
ventral anterior cingulate cortex (vACC): located in the ventral region of the anterior cingulate cortex, the ventral anterior cingulate cortex includes parts of Brodmann areas 24, 25, and 33. It is involved in emotional regulation, attention, and pain processing.
ventral entorhinal cortex (VEC): situated in the medial temporal lobe, the ventral entorhinal cortex covers parts of Brodmann area 28. It is involved in object recognition, memory, and contextual processing.
ventral posterior cingulate cortex (vPCC): located in the ventral part of the posterior cingulate cortex, the ventral posterior cingulate cortex includes parts of Brodmann areas 23 and 31. It involves self-referential thought, episodic memory retrieval, and emotional processing. ventromedial frontal cortex: a region of the frontal lobes involved in emotion regulation, decision-making, and social behavior, closely associated with the limbic system.
ventromedial orbitofrontal cortex: a subregion of the orbitofrontal cortex involved in processing reward-related information and decision-making.
visual association cortex: found in the occipital and parietal lobes, the visual association cortex includes Brodmann areas 18, 19, and parts of 7. It is responsible for higher-level visual processing, including object recognition, motion perception, and spatial awareness.
References
Aalst, J., Devrome, M., Weehaeghe, D., Rezaei, A., Radwan, A., Schramm, G., Ceccarini, J., Sunaert, S., Koole, M., & Laere, K. (2021). Regional glucose metabolic decreases with ageing are associated with microstructural white matter changes: a simultaneous PET/MR study. European Journal of Nuclear Medicine and Molecular Imaging. https://doi.org/10.1007/s00259-021-05518-6 Åberg, K., Dean, B., Shabalin, A., Chan, R., Han, L., Zhao, M., Grootheest, G., Xie, L., Milaneschi, Y., Clark, S., Turecki, G., Penninx, B., & Oord, E. (2018). Methylome-wide association findings for major depressive disorder overlap in blood and brain and replicate in independent brain samples. Molecular Psychiatry, 25, 1344 - 1354. https://doi.org/10.1038/s41380-018-0247-6 Aggleton, J. P., & Brown, M. W. (1999). Episodic memory, amnesia, and the hippocampal-anterior thalamic axis. The Behavioral and Brain Sciences, 22(3), 425–489.
Ahuja, A., & Yusif Rodriguez, N. (2022). Is the dorsolateral prefrontal cortex actually several different brain areas? The Journal of Neuroscience: The Official Journal of the Society for Neuroscience, 42(33), 6310–6312. https://doi.org/10.1523/JNEUROSCI.0848-22.2022
Almeida, J., Kronhaus, D., Sibille, E., Langenecker, S., Versace, A., LaBarbara, E., & Phillips, M. (2011). Abnormal left-sided orbitomedial prefrontal cortical–amygdala connectivity during happy and fear face processing: A potential neural mechanism of female MDD. Frontiers in Psychiatry, 2. https://doi.org/10.3389/fpsyt.2011.00069
Amaral, D. G., & Witter, M. P. (1995). Hippocampal formation. In G. Paxinos (Ed.), The rat nervous system (pp. 443-493). Academic Press.
Amunts, K., Malikovic, A., Mohlberg, H., Schormann, T., & Zilles, K. (2000). Brodmann's Areas 17 and 18 brought into stereotaxic space—Where and how variable? NeuroImage, 11, 66-84. https://doi.org/10.1006/nimg.1999.0516
Amunts, K., Schleicher, A., Bürgel, U., Mohlberg, H., Uylings, H. B., & Zilles, K. (1999). Broca's region revisited: Cytoarchitecture and intersubject variability. The Journal of Comparative Neurology, 412(2), 319–341. https://doi.org/10.1002/(sici)1096-9861(19990920)412:2<319::aid-cne10>3.0.co;2-7
Amunts, K., & Zilles, K. (2015). Architectonic mapping of the human brain beyond Brodmann. Neuron, 88, 1086-1107. https://doi.org/10.1016/j.neuron.2015.12.001
Ardila, A., Bernal, B., & Rosselli, M. (2015). Language and visual perception associations: Meta-analytic connectivity modeling of Brodmann area 37. Behavioural Neurology, 2015, 565871. https://doi.org/10.1155/2015/565871
Augustinack, J., Huber, K., Stevens, A., Roy, M., Frosch, M., Kouwe, A., Wald, L., Leemput, K., Mckee, A., & Fischl, B. (2013). Predicting the location of human perirhinal cortex, Brodmann's area 35, from MRI. NeuroImage, 64, 32-42. https://doi.org/10.1016/j.neuroimage.2012.08.071
Augustine J. R. (1996). Circuitry and functional aspects of the insular lobe in primates including humans. Brain Research. Brain Research Reviews, 22(3), 229–244. https://doi.org/10.1016/s0165-0173(96)00011-2
Avidan, G., & Behrmann, M. (2009). Functional MRI reveals compromised neural integrity of the face processing network in congenital prosopagnosia. Current Biology: CB, 19(13), 1146–1150. https://doi.org/10.1016/j.cub.2009.04.060
Baliki, M. N., Geha, P. Y., & Apkarian, A. V. (2009). Parsing pain perception between nociceptive representation and magnitude estimation. Journal of Neurophysiology, 101(2), 875–887. https://doi.org/10.1152/jn.91100.2008
Bizley, J. K., & Cohen, Y. E. (2013). The what, where and how of auditory-object perception. Nature Reviews. Neuroscience, 14(10), 693–707. https://doi.org/10.1038/nrn3565
Booth, J. R., Wood, L., Lu, D., Houk, J. C., & Bitan, T. (2007). The role of the basal ganglia and cerebellum in language processing. Brain Research, 1133(1), 136–144. https://doi.org/10.1016/j.brainres.2006.11.074
Braak, H., & Braak, E. (1991). Neuropathological stageing of Alzheimer-related changes. Acta Neuropathologica, 82(4), 239–259. https://doi.org/10.1007/BF00308809
Bressler, S. L., & Menon, V. (2010). Large-scale brain networks in cognition: emerging methods and principles. Trends in Cognitive Sciences, 14(6), 277–290. https://doi.org/10.1016/j.tics.2010.04.004
Brodmann, K. (1909). Vergleichende Lokalisationslehre der Grosshirnrinde in ihren Prinzipien dargestellt auf Grund des Zellenbaues. Barth.
Broyd, S. J., Demanuele, C., Debener, S., Helps, S. K., James, C. J., & Sonuga-Barke, E. J. (2009). Default-mode brain dysfunction in mental disorders: A systematic review. Neuroscience and Biobehavioral Reviews, 33(3), 279–296. https://doi.org/10.1016/j.neubiorev.2008.09.002
Buckner, R. L., Andrews-Hanna, J. R., & Schacter, D. L. (2008). The brain's default network: Anatomy, function, and relevance to disease. Annals of the New York Academy of Sciences, 1124, 1-38. https://doi.org/10.1196/annals.1440.011
Bush, G., Luu, P., & Posner, M. I. (2000). Cognitive and emotional influences in anterior cingulate cortex. Trends in Cognitive Sciences, 4(6), 215–222. https://doi.org/10.1016/s1364-6613(00)01483-2
Cao, Z., Ottino-Gonzalez, J., Cupertino, R. B., Schwab, N., Hoke, C., Catherine, O., Cousijn, J., Dagher, A., Foxe, J. J., Goudriaan, A. E., Hester, R., Hutchison, K., Li, C. R., London, E. D., Lorenzetti, V., Luijten, M., Martin-Santos, R., Momenan, R., Paulus, M. P., Schmaal, L., … Garavan, H. (2021). Mapping cortical and subcortical asymmetries in substance dependence: Findings from the ENIGMA Addiction Working Group. Addiction Biology, 26(5), e13010. https://doi.org/10.1111/adb.13010
Carey, L. M., Abbott, D. F., Egan, G. F., O'Keefe, G. J., Jackson, G. D., Bernhardt, J., & Donnan, G. A. (2006). Evolution of brain activation with good and poor motor recovery after stroke. Neurorehabilitation and Neural Repair, 20(1), 24–41. https://doi.org/10.1177/1545968305283053
Carlén M. (2017). What constitutes the prefrontal cortex? Science, 358(6362), 478–482. https://doi.org/10.1126/science.aan8868
Caspers, S., Geyer, S., Schleicher, A., Mohlberg, H., Amunts, K., & Zilles, K. (2006). The human inferior parietal cortex: Cytoarchitectonic parcellation and interindividual variability. NeuroImage, 33(2), 430–448. https://doi.org/10.1016/j.neuroimage.2006.06.054
Castellanos, F. X., Margulies, D. S., Kelly, C., Uddin, L. Q., Ghaffari, M., Kirsch, A., Shaw, D., Shehzad, Z., Di Martino, A., Biswal, B., Sonuga-Barke, E. J., Rotrosen, J., Adler, L. A., & Milham, M. P. (2008). Cingulate-precuneus interactions: A new locus of dysfunction in adult attention-deficit/hyperactivity disorder. Biological Psychiatry, 63(3), 332–337. https://doi.org/10.1016/j.biopsych.2007.06.025
Catani, M., Jones, D. K., Donato, R., & Ffytche, D. H. (2003). Occipito-temporal connections in the human brain. Brain: A Journal of Neurology, 126(Pt 9), 2093–2107. https://doi.org/10.1093/brain/awg203
Chan, C., Szeszko, P., Wong, E., Tang, C., Kelliher, C., Penner, J., Perez-Rodriguez, M., Rosell, D., McClure, M., Roussos, P., New, A., Siever, L., & Hazlett, E. (2018). Frontal and temporal cortical volume, white matter tract integrity, and hemispheric asymmetry in schizotypal personality disorder. Schizophrenia Research, 197, 226-232. https://doi.org/10.1016/j.schres.2018.01.025
Chen, L., Sun, D., Davis, S., Haswell, C., Dennis, E., Swanson, C., Whelan, C., Gutman, B., Jahanshad, N., Iglesias, J., Thompson, P., Wagner, H., Saemann, P., LaBar, K., & Morey, R. (2018). Smaller hippocampal CA-1 subfield volume in Posttraumatic Stress Disorder. bioRxiv. https://doi.org/10.1002/da.22833
Cheng, W., Rolls, E., Qiu, J., Liu, W., Tang, Y., Huang, C., Wang, X., Zhang, J., Lin, W., Zheng, L., Pu, J., Tsai, S., Yang, A., Lin, C., Wang, F., Xie, P., & Feng, J. (2016). Medial reward and lateral non-reward orbitofrontal cortex circuits change in opposite directions in depression. Brain: A Journal of Neurology, 139(Pt 12), 3296-3309. https://doi.org/10.1093/BRAIN/AWW255
Craig A. D. (2009). How do you feel--now? The anterior insula and human awareness. Nature reviews. Neuroscience, 10(1), 59–70. https://doi.org/10.1038/nrn2555
Dean, B., Tawadros, N., Scarr, E., & Gibbons, A. (2010). Regionally-specific changes in levels of tumour necrosis factor in the dorsolateral prefrontal cortex obtained postmortem from subjects with major depressive disorder. Journal of Affective Disorders, 120(1-3), 245-8 . https://doi.org/10.1016/j.jad.2009.04.027
Deery, H., Paolo, R., Moran, C., Egan, G., & Jamadar, S. (2022). Lower brain glucose metabolism in normal ageing is predominantly frontal and temporal: A systematic review and pooled effect size and activation likelihood estimates meta‐analyses. Human Brain Mapping, 44, 1251 - 1277. https://doi.org/10.1002/hbm.26119
Devinsky, O., Morrell, M. J., & Vogt, B. A. (1995). Contributions of anterior cingulate cortex to behaviour. Brain: A Journal of Neurology, 118 ( Pt 1), 279–306. https://doi.org/10.1093/brain/118.1.279
Dosenbach, N. U., Fair, D. A., Miezin, F. M., Cohen, A. L., Wenger, K. K., Dosenbach, R. A., Fox, M. D., Snyder, A. Z., Vincent, J. L., Raichle, M. E., Schlaggar, B. L., & Petersen, S. E. (2007). Distinct brain networks for adaptive and stable task control in humans. Proceedings of the National Academy of Sciences of the United States of America, 104(26), 11073–11078. https://doi.org/10.1073/pnas.0704320104
Doty R. L. (2008). The olfactory vector hypothesis of neurodegenerative disease: is it viable? Annals of Neurology, 63(1), 7–15. https://doi.org/10.1002/ana.21327
Drevets, W. C., Price, J. L., & Furey, M. L. (2008). Brain structural and functional abnormalities in mood disorders: Implications for neurocircuitry models of depression. Brain Structure & Function, 213(1-2), 93–118. https://doi.org/10.1007/s00429-008-0189-x
Du, F., Whetsell, W. O., Jr, Abou-Khalil, B., Blumenkopf, B., Lothman, E. W., & Schwarcz, R. (1993). Preferential neuronal loss in layer III of the entorhinal cortex in patients with temporal lobe epilepsy. Epilepsy Research, 16(3), 223–233. https://doi.org/10.1016/0920-1211(93)90083-j
Eichenbaum, H., Yonelinas, A. P., & Ranganath, C. (2007). The medial temporal lobe and recognition memory. Annual Review of Neuroscience, 30, 123–152. https://doi.org/10.1146/annurev.neuro.30.051606.094328
Eifuku, S. (2017). [Brodmann areas 27, 28, 36 and 37: The parahippocampal and the fusiform gyri]. Brain and nerve = Shinkei kenkyu no shinpo, 69(4,) 439-451 . https://doi.org/10.11477/mf.1416200762
Ellison-Wright, I., & Bullmore, E. (2009). Meta-analysis of diffusion tensor imaging studies in schizophrenia. Schizophrenia Research, 108, 3-10. https://doi.org/10.1016/j.schres.2008.11.021
Epstein R. A. (2008). Parahippocampal and retrosplenial contributions to human spatial navigation. Trends in Cognitive Sciences, 12(10), 388–396. https://doi.org/10.1016/j.tics.2008.07.004
Etkin, A., Egner, T., & Kalisch, R. (2011). Emotional processing in anterior cingulate and medial prefrontal cortex. Trends in Cognitive Sciences, 15(2), 85–93. https://doi.org/10.1016/j.tics.2010.11.004
Etkin, A., & Wager, T. (2007). Functional neuroimaging of anxiety: a meta-analysis of emotional processing in PTSD, social anxiety disorder, and specific phobia. The American Journal of Psychiatry, 164(10), 1476-88. https://doi.org/10.1176/APPI.AJP.2007.07030504
Farré-Colomés, Á., Gerhardt, S., Luderer, M., Sobanski, E., Kiefer, F., & Vollstädt-Klein, S. (2021). Common and distinct neural connectivity in Attention Deficit/Hyperactivity Disorder and Alcohol Use Disorder: A study using resting-state functional magnetic resonance imaging. Alcoholism, Clinical and Experimental Research. https://doi.org/10.1111/acer.14593
Feldker, K., Heitmann, C., Neumeister, P., Bruchmann, M., Vibrans, L., Zwitserlood, P., & Straube, T. (2016). Brain responses to disorder‐related visual threat in panic disorder. Human Brain Mapping, 37. https://doi.org/10.1002/hbm.23320
Feldker, K., Heitmann, C., Neumeister, P., Brinkmann, L., Bruchmann, M., Zwitserlood, P., & Straube, T. (2017). Cardiorespiratory concerns shape brain responses during automatic panic-related scene processing in patients with panic disorder. Journal of Psychiatry & Neuroscience, 43, 26–36. https://doi.org/10.1503/jpn.160226
Flores, R., Das, S., Xie, L., Wisse, L., Lyu, X., Shah, P., Yushkevich, P., & Wolk, D. (2022). Medial temporal lobe networks in Alzheimer's disease: Structural and molecular vulnerabilities. The Journal of Neuroscience, 42, 2131 - 2141. https://doi.org/10.1523/JNEUROSCI.0949-21.2021
Frank, G. K., Shott, M. E., Riederer, J., & Pryor, T. L. (2016). Altered structural and effective connectivity in anorexia and bulimia nervosa in circuits that regulate energy and reward homeostasis. Translational Psychiatry, 6(11), e932. https://doi.org/10.1038/tp.2016.199
Friederici A. D. (2011). The brain basis of language processing: From structure to function. Physiological Reviews, 91(4), 1357–1392. https://doi.org/10.1152/physrev.00006.2011
Fuster J. M. (2001). The prefrontal cortex--an update: time is of the essence. Neuron, 30(2), 319–333. https://doi.org/10.1016/s0896-6273(01)00285-9
Gaddis, A., Rosch, K., Dirlikov, B., Crocetti, D., MacNeil, L., Barber, A., Muschelli, J., Caffo, B., Pekar, J., & Mostofsky, S. (2015). Motor overflow in children with attention-deficit/hyperactivity disorder is associated with decreased extent of neural activation in the motor cortex. Psychiatry Research: Neuroimaging, 233, 488-495. https://doi.org/10.1016/j.pscychresns.2015.08.001
Gao, Y., Wang, X., Xiong, Z., Ren, H., Liu, R., Wei, Y., & Li, D. (2021). Abnormal fractional amplitude of low-frequency fluctuation as a potential imaging biomarker for first-episode Major Depressive Disorder: A resting-state fMRI study and support vector machine analysis. Frontiers in Neurology, 12. https://doi.org/10.3389/fneur.2021.751400
Gardener, S., Sohrabi, H., Shen, K., Rainey-Smith, S., Weinborn, M., Bates, K., Shah, T., Foster, J., Lenzo, N., Salvado, O., Laske, C., Laws, S., Taddei, K., Verdile, G., & Martins, R. (2016). Cerebral glucose metabolism is associated with verbal but not visual memory performance in community-dwelling older adults. Journal of Alzheimer's Disease: JAD, 52(2), 661-72. https://doi.org/10.3233/JAD-151084.
Gevensleben, H., Moll, G. H., Rothenberger, A., & Heinrich, H. (2014). Neurofeedback in attention-deficit/hyperactivity disorder - Different models, different ways of application. Frontiers in Human Neuroscience, 8, 846. https://doi.org/10.3389/fnhum.2014.00846
Gilbert, D., Huddleston, D., Wu, S., Pedapati, E., Horn, P., Hirabayashi, K., Crocetti, D., Wassermann, E., & Mostofsky, S. (2019). Motor cortex inhibition and modulation in children with ADHD. Neurology, 93, e599 - e610. https://doi.org/10.1212/WNL.0000000000007899
Gilbert, D., Isaacs, K., Augusta, M., MacNeil, L., & Mostofsky, S. (2011). Motor cortex inhibition. Neurology, 76, 615 - 621. https://doi.org/10.1212/WNL.0b013e31820c2ebd
Glasser, M. F., Coalson, T. S., Robinson, E. C., Hacker, C. D., Harwell, J., Yacoub, E., Ugurbil, K., Andersson, J., Beckmann, C. F., Jenkinson, M., Smith, S. M., & Van Essen, D. C. (2016). A multi-modal parcellation of human cerebral cortex. Nature, 536(7615), 171–178. https://doi.org/10.1038/nature18933
Goldsmith, S., Shapiro, R., & Joyce, J. (1997). Disrupted pattern of D2 dopamine receptors in the temporal lobe in schizophrenia. A postmortem study. Archives of General Psychiatry, 54(7), 649-58. https://doi.org/10.1001/ARCHPSYC.1997.01830190077008
Gottfried J. A. (2010). Central mechanisms of odour object perception. Nature Reviews. Neuroscience, 11(9), 628–641. https://doi.org/10.1038/nrn2883
Greicius, M. D., Flores, B. H., Menon, V., Glover, G. H., Solvason, H. B., Kenna, H., Reiss, A. L., & Schatzberg, A. F. (2007). Resting-state functional connectivity in major depression: Abnormally increased contributions from subgenual cingulate cortex and thalamus. Biological Psychiatry, 62(5), 429–437. https://doi.org/10.1016/j.biopsych.2006.09.020
Griffiths, T. D., & Warren, J. D. (2002). The planum temporale as a computational hub. Trends in Neurosciences, 25(7), 348–353. https://doi.org/10.1016/s0166-2236(02)02191-4
Grill-Spector, K., Knouf, N., & Kanwisher, N. (2004). The fusiform face area subserves face perception, not generic within-category identification. Nature Neuroscience, 7(5), 555–562. https://doi.org/10.1038/nn1224
Hafting, T., Fyhn, M., Molden, S., Moser, M. B., & Moser, E. I. (2005). Microstructure of a spatial map in the entorhinal cortex. Nature, 436(7052), 801–806. https://doi.org/10.1038/nature03721
Hamilton, J. P., Glover, G. H., Hsu, J. J., Johnson, R. F., & Gotlib, I. H. (2011). Modulation of subgenual anterior cingulate cortex activity with real-time neurofeedback. Human Brain Mapping, 32(1), 22–31. https://doi.org/10.1002/hbm.20997
Harricharan, S., Nicholson, A., Thome, J., Densmore, M., McKinnon, M., Théberge, J., Frewen, P., Neufeld, R., & Lanius, R. (2019). PTSD and its dissociative subtype through the lens of the insula: Anterior and posterior insula resting-state functional connectivity and its predictive validity using machine learning. Psychophysiology, e13472 . https://doi.org/10.1111/psyp.13472
Hasler, G., Fromm, S., Alvarez, R., Luckenbaugh, D., Drevets, W., & Grillon, C. (2007). Cerebral blood flow in immediate and sustained anxiety. The Journal of Neuroscience, 27, 6313 - 6319. https://doi.org/10.1523/JNEUROSCI.5369-06.2007
Hazlett, E., Buchsbaum, M., Hsieh, P., Haznedar, M., Platholi, J., LiCalzi, E., Cartwright, C., & Hollander, E. (2004). Regional glucose metabolism within cortical Brodmann areas in healthy individuals and autistic patients. Neuropsychobiology, 49, 115 - 125. https://doi.org/10.1159/000076719
Hazlett, E., Byne, W., Brickman, A., Brickman, A., Mitsis, E., Newmark, R., Haznedar, M., Knatz, D., Chen, A., & Buchsbaum, M. (2010). Effects of sex and normal aging on regional brain activation during verbal memory performance. Neurobiology of Aging, 31, 826-838. https://doi.org/10.1016/j.neurobiolaging.2008.10.005
Hazlett, E., Goldstein, K., Tajima-Pozo, K., Speidel, E., Zelmanova, Y., Entis, J., Silverman, J., New, A., Koenigsberg, H., Haznedar, M., Byne, W., & Siever, L. (2011). Cingulate and temporal lobe fractional anisotropy in schizotypal personality disorder. NeuroImage, 55, 900-908. https://doi.org/10.1016/j.neuroimage.2010.12.082
Heckers, S., Rauch, S. L., Goff, D., Savage, C. R., Schacter, D. L., Fischman, A. J., & Alpert, N. M. (1998). Impaired recruitment of the hippocampus during conscious recollection in schizophrenia. Nature Neuroscience, 1(4), 318–323. https://doi.org/10.1038/1137
Herringa, R., Phillips, M., Fournier, J., Kronhaus, D., & Germain, A. (2012). Childhood and adult trauma both correlate with dorsal anterior cingulate activation to threat in combat veterans. Psychological Medicine, 43, 1533 - 1542. https://doi.org/10.1017/S0033291712002310
Ho, A., Winham, S., Armasu, S., Blacker, C., Millischer, V., Lavebratt, C., Overholser, J., Jurjus, G., Dieter, L., Mahajan, G., Rajkowska, G., Vallender, E., Stockmeier, C., Robertson, K., Frye, M., Choi, D., & Veldic, M. (2019). Genome-wide DNA methylomic differences between dorsolateral prefrontal and temporal pole cortices of bipolar disorder. Journal of Psychiatric Research, 117, 45-54. https://doi.org/10.1016/j.jpsychires.2019.05.030
Hoeft, F., Meyler, A., Hernandez, A., Juel, C., Taylor-Hill, H., Martindale, J. L., McMillon, G., Kolchugina, G., Black, J. M., Faizi, A., Deutsch, G. K., Siok, W. T., Reiss, A. L., Whitfield-Gabrieli, S., & Gabrieli, J. D. (2007). Functional and morphometric brain dissociation between dyslexia and reading ability. Proceedings of the National Academy of Sciences of the United States of America, 104(10), 4234–4239. https://doi.org/10.1073/pnas.0609399104
Hoesen, G., Hyman, B., & Damasio, A. (1991). Entorhinal cortex pathology in Alzheimer's disease. Hippocampus, 1. https://doi.org/10.1002/hipo.450010102
Hoogman, M., Muetzel, R., Guimaraes, J., Shumskaya, E., Mennes, M., Zwiers, M., Jahanshad, N., Sudre, G., Wolfers, T., Earl, E., Vila, J., Vives-Gilabert, Y., Khadka, S., Novotny, S., Hartman, C., Heslenfeld, D., Schweren, L., Ambrosino, S., ... Franke, B. (2019). Brain imaging of the cortex in ADHD: A coordinated analysis of large-scale clinical and population-based samples. The American Journal of Psychiatry, 176(7), 531-542. https://doi.org/10.1176/appi.ajp.2019.18091033
Hoshi, E., & Tanji, J. (2007). Distinctions between dorsal and ventral premotor areas: Anatomical connectivity and functional properties. Current Opinion in Neurobiology, 17, 234-242. https://doi.org/10.1016/j.conb.2007.02.003
Hull, A. (2002). Neuroimaging findings in post-traumatic stress disorder. British Journal of Psychiatry, 181, 102 - 110. https://doi.org/10.1192/bjp.181.2.102
Hyman, B. T., Van Hoesen, G. W., Damasio, A. R., & Barnes, C. L. (1984). Alzheimer's disease: Cell-specific pathology isolates the hippocampal formation. Science, 225(4667), 1168–1170. https://doi.org/10.1126/science.6474172
Jacot-Descombes, S., Uppal, N., Wicinski, B., Santos, M., Schmeidler, J., Giannakopoulos, P., Heinsein, H., Schmitz, C., & Hof, P. (2012). Decreased pyramidal neuron size in Brodmann areas 44 and 45 in patients with autism. Acta Neuropathologica, 124, 67-79. https://doi.org/10.1007/s00401-012-0976-6
Jasper, H. H. (1958). The Ten-Twenty Electrode System of the International Federation. Electroencephalography and Clinical Neurophysiology, 10, 371-375.
Johnstone, T., Reekum, C., Urry, H., Kalin, N., & Davidson, R. (2007). Failure to regulate: Counterproductive recruitment of top-down prefrontal-subcortical circuitry in Major Depression. The Journal of Neuroscience, 27, 8877 - 8884. https://doi.org/10.1523/JNEUROSCI.2063-07.2007
Joyce, J., Goldsmith, S., & Gurevich, E. (1997). Limbic circuits and monoamine receptors: dissecting the effects of antipsychotics from disease processes. Journal of Psychiatric Research, 31(2), 197-217. https://doi.org/10.1016/S0022-3956(96)00043-X
Karalok, Z., Öztürk, Z., & Gunes, A. (2019). Cortical thinning in benign epilepsy with centrotemporal spikes (BECTS) with or without attention-deficit/hyperactivity (ADHD). Journal of Clinical Neuroscience, 68, 123-127. https://doi.org/10.1016/j.jocn.2019.07.014
Kaur, A., Basavanagowda, D., Rathod, B., Mishra, N., Fuad, S., Nosher, S., Alrashid, Z., Mohan, D., & Heindl, S. (2020). Structural and functional alterations of the temporal lobe in schizophrenia: A literature review. Cureus, 12. https://doi.org/10.7759/cureus.11177
Kawamura, M., Miller, M., Ichikawa, H., Ishihara, K., & Sugimoto, A. (2011). Brodmann area 12. Neurology, 76, 1596-1599. https://doi.org/10.1212/WNL.0b013e3182190cd8
Khan, U. A., Liu, L., Provenzano, F. A., Berman, D. E., Profaci, C. P., Sloan, R., Mayeux, R., Duff, K. E., & Small, S. A. (2014). Molecular drivers and cortical spread of lateral entorhinal cortex dysfunction in preclinical Alzheimer's disease. Nature Neuroscience, 17(2), 304–311. https://doi.org/10.1038/nn.3606
Kikuchi, M., Yoshimura, Y., Shitamichi, K., Ueno, S., Hiraishi, H., Munesue, T., Hirosawa, T., Ono, Y., Tsubokawa, T., Inoue, Y., Oi, M., Niida, Y., Remijn, G., Takahashi, T., Suzuki, M., Higashida, H., & Minabe, Y. (2013). Anterior prefrontal hemodynamic connectivity in conscious 3- to 7-year-old children with typical development and Autism Spectrum Disorder. PLoS ONE, 8. https://doi.org/10.1371/journal.pone.0056087
Kluetsch, R. C., Ros, T., Théberge, J., Frewen, P. A., Calhoun, V. D., Schmahl, C., Jetly, R., & Lanius, R. A. (2014). Plastic modulation of PTSD resting-state networks and subjective wellbeing by EEG neurofeedback. Acta Psychiatrica Scandinavica, 130(2), 123–136. https://doi.org/10.1111/acps.12229
Kringelbach M. L. (2005). The human orbitofrontal cortex: Linking reward to hedonic experience. Nature Reviews. Neuroscience, 6(9), 691–702. https://doi.org/10.1038/nrn1747
Kurth, F., Zilles, K., Fox, P. T., Laird, A. R., & Eickhoff, S. B. (2010). A link between the systems: Functional differentiation and integration within the human insula revealed by meta-analysis. Brain Structure & Function, 214(5-6), 519–534. https://doi.org/10.1007/s00429-010-0255-z
Lake, E., Finn, E., Noble, S., Vanderwal, T., Shen, X., Rosenberg, M., Rosenberg, M., Spann, M., Chun, M., Scheinost, D., & Constable, R. (2019). The functional brain organization of an individual allows prediction of measures of social abilities transdiagnostically in autism and Attention-Deficit/Hyperactivity Disorder. Biological Psychiatry, 86, 315-326. https://doi.org/10.1016/j.biopsych.2019.02.019
Lai, C., & Wu, Y. (2015). The patterns of fractional amplitude of low-frequency fluctuations in depression patients: The dissociation between temporal regions and fronto-parietal regions. Journal of Affective Disorders, 175, 441-445. https://doi.org/10.1016/j.jad.2015.01.054
Lee, T., Kim, S., & Kwon, J. (2016). Disrupted thalamo-orbitofrontal but not fronto-temporal white matter connectivity in people with schizotypal personality disorder. European Psychiatry, 33, S103 - S103. https://doi.org/10.1016/j.eurpsy.2016.01.082
Leech, R., & Sharp, D. J. (2014). The role of the posterior cingulate cortex in cognition and disease. Brain: A Journal of Neurology, 137(Pt 1), 12–32. https://doi.org/10.1093/brain/awt162
Leuba, G., Savioz, A., Vernay, A., Carnal, B., Kraftsik, R., Tardif, E., Riederer, I., & Riederer, B. (2008). Differential changes in synaptic proteins in the Alzheimer frontal cortex with marked increase in PSD-95 postsynaptic protein. Journal of Alzheimer's Disease: JAD, 15(1), 139-51 . https://doi.org/10.3233/JAD-2008-15112
Linden D. E. (2006). How psychotherapy changes the brain--The contribution of functional neuroimaging. Molecular Psychiatry, 11(6), 528–538. https://doi.org/10.1038/sj.mp.4001816
Liston, C., Malter Cohen, M., Teslovich, T., Levenson, D., & Casey, B. J. (2011). Atypical prefrontal connectivity in attention-deficit/hyperactivity disorder: Pathway to disease or pathological end point? Biological Psychiatry, 69(12), 1168–1177. https://doi.org/10.1016/j.biopsych.2011.03.022
Manthey, A., Sierk, A., Brakemeier, E., Walter, H., & Daniels, J. (2021). Does trauma-focused psychotherapy change the brain? A systematic review of neural correlates of therapeutic gains in PTSD. European Journal of Psychotraumatology, 12. https://doi.org/10.1080/20008198.2021.1929025
Margulies, D. S., Kelly, A. M., Uddin, L. Q., Biswal, B. B., Castellanos, F. X., & Milham, M. P. (2007). Mapping the functional connectivity of anterior cingulate cortex. NeuroImage, 37(2), 579–588. https://doi.org/10.1016/j.neuroimage.2007.05.019
Mathew, I., Gardin, T., Tandon, N., Eack, S., Francis, A., Seidman, L., Clementz, B., Pearlson, G., Sweeney, J., Tamminga, C., & Keshavan, M. (2014). Medial temporal lobe structures and hippocampal subfields in psychotic disorders: findings from the Bipolar-Schizophrenia Network on Intermediate Phenotypes (B-SNIP) study. JAMA Psychiatry, 71 7, 769-77. https://doi.org/10.1001/jamapsychiatry.2014.453
Mayberg H. S. (2003). Modulating dysfunctional limbic-cortical circuits in depression: Towards development of brain-based algorithms for diagnosis and optimised treatment. British Medical Bulletin, 65, 193–207. https://doi.org/10.1093/bmb/65.1.193
McCarley, R., Wible, C., Frumin, M., Hirayasu, Y., Levitt, J., Fischer, I., & Shenton, M. (1999). MRI anatomy of schizophrenia. Biological Psychiatry, 45, 1099-1119. https://doi.org/10.1016/S0006-3223(99)00018-9
Mckee, A., Au, R., Cabral, H., Kowall, N., Seshadri, S., Kubilus, C., Drake, J., & Wolf, P. (2006). Visual association pathology in preclinical Alzheimer disease. Journal of Neuropathology and Experimental Neurology, 65, 621-630. https://doi.org/10.1097/00005072-200606000-00010
McLaughlin, K., Sheridan, M., Winter, W., Fox, N., Zeanah, C., & Nelson, C. (2014). Widespread reductions in cortical thickness following severe early-life deprivation: A neurodevelopmental pathway to Attention-Deficit/Hyperactivity Disorder. Biological Psychiatry, 76, 629-638. https://doi.org/10.1016/j.biopsych.2013.08.016
Menon, V. (2011). Large-scale brain networks and psychopathology: A unifying triple network model. Trends in Cognitive Sciences, 15(10), 483-506. https://doi.org/10.1016/j.tics.2011.08.003
Menon, V., & Uddin, L. Q. (2010). Saliency, switching, attention and control: A network model of insula function. Brain Structure & Function, 214(5-6), 655–667. https://doi.org/10.1007/s00429-010-0262-0
Miyazawa, N., & Shinohara, T. (2022). Cerebral blood flow-single-photon emission computed tomography and Brodmann mapping may facilitate the evaluation of clinical features of early-onset Alzheimer’s disease. Frontiers in Clinical Neurology and Neurosurgery. https://doi.org/10.36879/fcnn.2022.000101.
Morosan, P., Rademacher, J., Schleicher, A., Amunts, K., Schormann, T., & Zilles, K. (2001). Human primary auditory cortex: Cytoarchitectonic subdivisions and mapping into a spatial reference system. NeuroImage, 13(4), 684–701. https://doi.org/10.1006/nimg.2000.0715
Mummery, C., Patterson, K., Price, C., Ashburner, J., Frackowiak, R., & Hodges, J. (2000). A voxel‐based morphometry study of semantic dementia: Relationship between temporal lobe atrophy and semantic memory. Annals of Neurology, 47.
https://doi.org/10.1002/1531-8249(200001)47:1<36::AID-ANA8>3.0.CO;2-L
Mummery, C., Patterson, K., Price, C., & Hodges, J. (1998). Disrupted temporal lobe connections in semantic dementia. NeuroImage, 7. https://doi.org/10.1016/S1053-8119(18)31038-3
Nakatani, N., Hattori, E., Ohnishi, T., Dean, B., Iwayama, Y., Matsumoto, I., Kato, T., Osumi, N., Higuchi, T., Niwa, S., & Yoshikawa, T. (2006). Genome-wide expression analysis detects eight genes with robust alterations specific to bipolar I disorder: Relevance to neuronal network perturbation. Human Molecular Genetics, 15(12), 1949-62 . https://doi.org/10.1093/HMG/DDL118
Nardone, S., Sams, D., Reuveni, E., Getselter, D., Oron, O., Karpuj, M., & Elliott, E. (2014). DNA methylation analysis of the autistic brain reveals multiple dysregulated biological pathways. Translational Psychiatry, 4. https://doi.org/10.1038/tp.2014.70
Neef, N. E., Anwander, A., Bütfering, C., Schmidt-Samoa, C., Friederici, A. D., Paulus, W., & Sommer, M. (2018). Structural connectivity of right frontal hyperactive areas scales with stuttering severity. Brain: A Journal of Neurology, 141(1), 191–204. https://doi.org/10.1093/brain/awx316
Nelson, P., Abner, E., Scheff, S., Schmitt, F., Kryscio, R., Jicha, G., Smith, C., Patel, E., & Markesbery, W. (2009). Alzheimer's-type neuropathology in the precuneus is not increased relative to other areas of neocortex across a range of cognitive impairment. Neuroscience Letters, 450, 336-339. https://doi.org/10.1016/j.neulet.2008.11.006
Neville, K. R., & Haberly, L. B. (2004). Olfactory cortex. The synaptic organization of the brain, In G. M. Shepherd (Ed.). Oxford University Press, 415–454. https://doi.org/10.1093/acprof:oso/9780195159561.003.0010
Niendam, T. A., Laird, A. R., Ray, K. L., Dean, Y. M., Glahn, D. C., & Carter, C. S. (2012). Meta-analytic evidence for a superordinate cognitive control network subserving diverse executive functions. Cognitive, Affective & Behavioral Neuroscience, 12(2), 241–268. https://doi.org/10.3758/s13415-011-0083-5
Niida, R., & Mimura, M. (2017). [The Center of Sadness, Pain, and Recognition].Brain and nerve = Shinkei kenkyu no shinpo, 69(4), 417-426 . https://doi.org/10.11477/mf.1416200759
Ogawa H. (1994). Gustatory cortex of primates: anatomy and physiology. Neuroscience Research, 20(1), 1–13. https://doi.org/10.1016/0168-0102(94)90017-5
Okamoto, H., Onitsuka, T., Kuga, H., Oribe, N., Nakayama, N., Fukushima, S., Nakao, T., & Ueno, T. (2022). Decreased BOLD signals elicited by 40-Hz auditory stimulation of the right primary auditory cortex in bipolar disorder: An fMRI study. Frontiers in Psychiatry, 13. https://doi.org/10.3389/fpsyt.2022.833896
Olabi, B., Ellison-Wright, I., McIntosh, A., Wood, S., Bullmore, E., & Lawrie, S. (2011). Are there progressive brain changes in schizophrenia? A meta-analysis of structural magnetic resonance imaging studies. Biological Psychiatry, 70, 88-96. https://doi.org/10.1016/j.biopsych.2011.01.032
Oldehinkel, M., Beckmann, C., Pruim, R., Oort, E., Franke, B., Hartman, C., Hoekstra, P., Oosterlaan, J., Heslenfeld, D., Buitelaar, J., & Mennes, M. (2016). Attention-Deficit/Hyperactivity Disorder symptoms coincide with altered striatal connectivity. Biological Psychiatry. Cognitive Neuroscience and Neuroimaging, 14, 353-363 . https://doi.org/10.1016/J.BPSC.2016.03.008
Olson, I. R., Plotzker, A., & Ezzyat, Y. (2007). The Enigmatic temporal pole: A review of findings on social and emotional processing. Brain: A Journal of Neurology, 130(Pt 7), 1718–1731. https://doi.org/10.1093/brain/awm052
Öngür, D., Drevets, W., & Price, J. (1998). Glial reduction in the subgenual prefrontal cortex in mood disorders. Proceedings of the National Academy of Sciences of the United States of America, 95(22), 13290-5. https://doi.org/10.1073/PNAS.95.22.13290
Ongür, D., Ferry, A. T., & Price, J. L. (2003). Architectonic subdivision of the human orbital and medial prefrontal cortex. The Journal of Comparative Neurology, 460(3), 425–449. https://doi.org/10.1002/cne.10609
Overmeyer, S., Bullmore, E., Suckling, J., Simmons, A., Williams, S., Santosh, P., & Taylor, E. (2001). Distributed grey and white matter deficits in hyperkinetic disorder: MRI evidence for anatomical abnormality in an attentional network. Psychological Medicine, 31, 1425 - 1435. https://doi.org/10.1017/S0033291701004706
Padmanabhan, A., Lynch, C. J., Schaer, M., & Menon, V. (2017). The Default Mode Network in autism. Biological Psychiatry. Cognitive Neuroscience and Neuroimaging, 2(6), 476–486. https://doi.org/10.1016/j.bpsc.2017.04.004
Palumbo, B., Siepi, D., Amici, S., & Sinzinger, H. (2014). Differential diagnosis between neurodegenerative dementia disorders and Parkinson’s disease using 99mTc-HMPAO SPECT. The Open Nuclear Medicine Journal, 6, 1-5. https://doi.org/10.2174/1876388X01406010001
Peng, K., Steele, S. C., Becerra, L., & Borsook, D. (2018). Brodmann area 10: Collating, integrating and high level processing of nociception and pain. Progress in Neurobiology, 161, 1–22. https://doi.org/10.1016/j.pneurobio.2017.11.004
Peng, X., Wu, X., Gong, R., Yang, R., Wang, X., Zhu, W., & Lin, P. (2020). Sub-regional anterior cingulate cortex functional connectivity revealed default network subsystem dysfunction in patients with major depressive disorder. Psychological Medicine, 51, 1687 - 1695. https://doi.org/10.1017/S0033291720000434
Pikusa, M., & Jończyk, R. (2015). Functional abnormalities in Broca’s area in adolescents with ADHD: A resting-state fMRI study. Poznan Studies in Contemporary Linguistics, 51, 163 - 177. https://doi.org/10.1515/psicl-2015-0006
Pissiota, A., Frans, Ö., Fernández, M., Knorring, L., Fischer, H., & Fredrikson, M. (2000). Neurofunctional correlates of posttraumatic stress disorder: A PET symptom provocation study. European Archives of Psychiatry and Clinical Neuroscience, 252, 68-75. https://doi.org/10.1007/s004060200014
Price, J. L., & Drevets, W. C. (2010). Neurocircuitry of mood disorders. Neuropsychopharmacology: Official Publication of the American College of Neuropsychopharmacology, 35(1), 192–216. https://doi.org/10.1038/npp.2009.104
Purves, D. (2018). Neuroscience (6th ed.). Oxford University Press.
Rabinak, C., Angstadt, M., Welsh, R., Kenndy, A., Lyubkin, M., Martis, B., & Phan, K. (2011). Altered amygdala resting-state functional connectivity in Post-Traumatic Stress Disorder. Frontiers in Psychiatry, 2. https://doi.org/10.3389/fpsyt.2011.00062
Raichle, M. E., MacLeod, A. M., Snyder, A. Z., Powers, W. J., Gusnard, D. A., & Shulman, G. L. (2001). A default mode of brain function. Proceedings of the National Academy of Sciences of the United States of America, 98(2), 676–682. https://doi.org/10.1073/pnas.98.2.676
Rajkowska, G., & Goldman-Rakic, P. S. (1995). Cytoarchitectonic definition of prefrontal areas in the normal human cortex: II. Variability in locations of areas 9 and 46 and relationship to the Talairach Coordinate System. Cerebral Cortex (1991), 5(4), 323–337. https://doi.org/10.1093/cercor/5.4.323
Ramnani, N., & Owen, A. M. (2004). Anterior prefrontal cortex: Insights into function from anatomy and neuroimaging. Nature Reviews. Neuroscience, 5(3), 184–194. https://doi.org/10.1038/nrn1343
Rolls E. T. (2006). Brain mechanisms underlying flavour and appetite. Philosophical Transactions of the Royal Society of London. Series B, Biological Sciences, 361(1471), 1123–1136. https://doi.org/10.1098/rstb.2006.1852
Rolls, E. T., Cheng, W., & Feng, J. (2020). The orbitofrontal cortex: Reward, emotion and depression. Brain Communications, 2(2), fcaa196. https://doi.org/10.1093/braincomms/fcaa196
Ros, T., Baars, B. J., Lanius, R. A., & Vuilleumier, P. (2014). Tuning pathological brain oscillations with neurofeedback: A systems neuroscience framework. Frontiers in Human Neuroscience, 8, 1008. https://doi.org/10.3389/fnhum.2014.01008
Roy, A. K., Shehzad, Z., Margulies, D. S., Kelly, A. M., Uddin, L. Q., Gotimer, K., Biswal, B. B., Castellanos, F. X., & Milham, M. P. (2009). Functional connectivity of the human amygdala using resting state fMRI. NeuroImage, 45(2), 614–626. https://doi.org/10.1016/j.neuroimage.2008.11.030
Roy, M., Shohamy, D., & Wager, T. D. (2012). Ventromedial prefrontal-subcortical systems and the generation of affective meaning. Trends in Cognitive Sciences, 16(3), 147–156. https://doi.org/10.1016/j.tics.2012.01.005
Rudebeck, P. H., Putnam, P. T., Daniels, T. E., Yang, T., Mitz, A. R., Rhodes, S. E., & Murray, E. A. (2014). A role for primate subgenual cingulate cortex in sustaining autonomic arousal. Proceedings of the National Academy of Sciences of the United States of America, 111(14), 5391–5396. https://doi.org/10.1073/pnas.1317695111
Sartory, G., Cwik, J., Knuppertz, H., Schürholt, B., Lebens, M., Seitz, R., & Schulze, R. (2013). In search of the trauma memory: A meta-analysis of functional neuroimaging studies of symptom provocation in Posttraumatic Stress Disorder (PTSD). PLoS ONE, 8. https://doi.org/10.1371/journal.pone.0058150
Scarr, E., Udawela, M., & Dean, B. (2019). Changed cortical risk gene expression in major depression and shared changes in cortical gene expression between major depression and bipolar disorders. Australian & New Zealand Journal of Psychiatry, 53, 1189 - 1198. https://doi.org/10.1177/0004867419857808
Sedley, W., Parikh, J., Edden, R. A., Tait, V., Blamire, A., & Griffiths, T. D. (2015). Human auditory cortex neurochemistry reflects the presence and severity of tinnitus. The Journal of Neuroscience: The Official Journal of the Society for Neuroscience, 35(44), 14822–14828. https://doi.org/10.1523/JNEUROSCI.2695-15.2015
Seeley, W. W., Menon, V., Schatzberg, A. F., Keller, J., Glover, G. H., Kenna, H., Reiss, A. L., & Greicius, M. D. (2007). Dissociable intrinsic connectivity networks for salience processing and executive control. The Journal of Neuroscience: The Official Journal of the Society for Neuroscience, 27(9), 2349–2356. https://doi.org/10.1523/JNEUROSCI.5587-06.2007
Seghier M. L. (2013). The angular gyrus: Multiple functions and multiple subdivisions. The Neuroscientist: A Review Journal Bridging Neurobiology, Neurology and Psychiatry, 19(1), 43–61. https://doi.org/10.1177/1073858412440596
Shang, J., Fu, Y., Ren, Z., Zhang, T., Du, M., Gong, Q., Lui, S., & Zhang, W. (2014). The Common Traits of the ACC and PFC in anxiety disorders in the DSM-5: Meta-analysis of voxel-based morphometry studies. PLoS ONE, 9. https://doi.org/10.1371/journal.pone.0093432
Sheline, Y. I., Price, J. L., Yan, Z., & Mintun, M. A. (2010). Resting-state functional MRI in depression unmasks increased connectivity between networks via the dorsal nexus. Proceedings of the National Academy of Sciences of the United States of America, 107(24), 11020–11025. https://doi.org/10.1073/pnas.1000446107
Shenton, M., Kikinis, R., Jolesz, F., Pollak, S., LeMay, M., Wible, C., Hokama, H., Martin, J., Metcalf, D., Coleman, M., & McCarley, R. (1992). Abnormalities of the left temporal lobe and thought disorder in schizophrenia. A quantitative magnetic resonance imaging study. The New England Journal of Medicine, 327(9), 604-12 . https://doi.org/10.1056/NEJM199208273270905.
Shepherd G. M. (2007). Perspectives on olfactory processing, conscious perception, and orbitofrontal cortex. Annals of the New York Academy of Sciences, 1121, 87–101. https://doi.org/10.1196/annals.1401.032
Silk, T., Vance, A., Rinehart, N., Egan, G., O'Boyle, M., Bradshaw, J., & Cunnington, R. (2005). Fronto-parietal activation in attention-deficit hyperactivity disorder, combined type: Functional magnetic resonance imaging study. The British Journal of Psychiatry: The Journal of Mental Science, 187, 282-3 . https://doi.org/10.1192/BJP.187.3.282
Small, D. M., Zald, D. H., Jones-Gotman, M., Zatorre, R. J., Pardo, J. V., Frey, S., & Petrides, M. (1999). Human cortical gustatory areas: A review of functional neuroimaging data. Neuroreport, 10(1), 7–14. https://doi.org/10.1097/00001756-199901180-00002
Sörös, P., Hoxhaj, E., Borel, P., Sadohara, C., Feige, B., Matthies, S., Müller, H., Bachmann, K., Schulze, M., & Philipsen, A. (2019). Hyperactivity/restlessness is associated with increased functional connectivity in adults with ADHD: A dimensional analysis of resting state fMRI. BMC Psychiatry, 19. https://doi.org/10.1186/s12888-019-2031-9
Speer, A., Willis, M., Herscovitch, P., Daube-Witherspoon, M., Shelton, J., Benson, B., Post, R., & Wassermann, E. (2003). Intensity-dependent regional cerebral blood flow during 1-Hz repetitive transcranial magnetic stimulation (rTMS) in healthy volunteers studied with h2 15o positron emission tomography: II. Effects of prefrontal cortex rTMS. Biological Psychiatry, 54, 826-832. https://doi.org/10.1016/S0006-3223(03)00324-X
Sripada, R., King, A., Garfinkel, S., Wang, X., Sripada, C., Welsh, R., & Liberzon, I. (2012). Altered resting-state amygdala functional connectivity in men with posttraumatic stress disorder. Journal of Psychiatry & Neuroscience: JPN, 37(4), 241-9. https://doi.org/10.1503/jpn.110069
Sultzer, D., Brown, C., Mandelkern, M., Mahler, M., Mendez, M., Chen, S., & Cummings, J. (2003). Delusional thoughts and regional frontal/temporal cortex metabolism in Alzheimer's disease. The American Journal of Psychiatry, 160(2), 341-9 . https://doi.org/10.1176/APPI.AJP.160.2.341
Suskauer, S., Simmonds, D., Caffo, B., Denckla, M., Pekar, J., & Mostofsky, S. (2008). fMRI of intrasubject variability in ADHD: anomalous premotor activity with prefrontal compensation. Journal of the American Academy of Child and Adolescent Psychiatry, 47(10), 1141-50 . https://doi.org/10.1097/CHI.0b013e3181825b1f
Suzuki, W. A., & Amaral, D. G. (1994). Perirhinal and parahippocampal cortices of the macaque monkey: Cortical afferents. The Journal of Comparative Neurology, 350(4), 497–533. https://doi.org/10.1002/cne.903500402
Szeszko, P., Robinson, D., Ashtari, M., Vogel, J., Betensky, J., Sevy, S., Ardekani, B., Lencz, T., Malhotra, A., McCormack, J., Miller, R., Lim, K., Gunduz-Bruce, H., Kane, J., & Bilder, R. (2008). Clinical and neuropsychological correlates of white matter abnormalities in recent onset schizophrenia. Neuropsychopharmacology, 33, 976-984. https://doi.org/10.1038/sj.npp.1301480
Thangavel, R., Sahu, S., Hoesen, G., & Zaheer, A. (2008). Modular and laminar pathology of Brodmann’s area 37 in Alzheimer’s disease. Neuroscience, 152, 50-55. https://doi.org/10.1016/j.neuroscience.2007.12.025
Tranfaglia, C., Palumbo, B., Siepi, D., Sinzinger, H., & Parnetti, L. (2009). Semi-quantitative analysis of perfusion of Brodmann areas in the differential diagnosis of cognitive impairment in Alzheimer's disease, fronto-temporal dementia and mild cognitive impairment. Hellenic Journal of Nuclear Medicine, 12(2), 110–114.
Utevsky, A. V., Smith, D. V., & Huettel, S. A. (2014). Precuneus is a functional core of the default-mode network. The Journal of Neuroscience: The Official Journal of the Society for Neuroscience, 34(3), 932–940. https://doi.org/10.1523/JNEUROSCI.4227-13.2014
Valotassiou, V., Papatriantafyllou, J., Sifakis, N., Tzavara, C., Tsougos, I., Kapsalaki, E., Hadjigeorgiou, G., & Georgoulias, P. (2012). Perfusion SPECT studies with mapping of Brodmann areas in differentiating Alzheimer’s disease from frontotemporal degeneration syndromes. Nuclear Medicine Communications, 33, 1267–1276. https://doi.org/10.1097/MNM.0b013e3283599983
Valotassiou, V., Papatriantafyllou, J., Sifakis, N., Tzavara, C., Tsougos, I., Psimadas, D., Fezoulidis, I., Kapsalaki, E., Hadjigeorgiou, G., & Georgoulias, P. (2015). Clinical evaluation of brain perfusion SPECT with Brodmann areas mapping in early diagnosis of Alzheimer's disease. Journal of Alzheimer's Disease: JAD, 47 (3), 773-785. https://doi.org/10.3233/JAD-150068
Valotassiou, V., Sifakis, N., Tzavara, C., Lykou, E., Tsinia, N., Kamtsadeli, V., Sali, D., Angelidis, G., Psimadas, D., Tsougos, I., Papageorgiou, S., Georgoulias, P., & Papatriantafyllou, J. (2021). Eating disorders in frontotemporal dementia and Alzheimer's disease: Evaluation of brain perfusion correlates using 99mTc-HMPAO SPECT with Brodmann areas analysis. Journal of Alzheimer's disease: JAD. https://doi.org/10.3233/JAD-201434
Van Hoesen, G., & Pandya, D. N. (1975). Some connections of the entorhinal (area 28) and perirhinal (area 35) cortices of the rhesus monkey. I. Temporal lobe afferents. Brain Research, 95(1), 1–24. https://doi.org/10.1016/0006-8993(75)90204-8
van Strien, N. M., Cappaert, N. L., & Witter, M. P. (2009). The anatomy of memory: An interactive overview of the parahippocampal-hippocampal network. Nature Reviews. Neuroscience, 10(4), 272–282. https://doi.org/10.1038/nrn2614
Vann, S. D., Aggleton, J. P., & Maguire, E. A. (2009). What does the retrosplenial cortex do? Nature Reviews. Neuroscience, 10(11), 792–802. https://doi.org/10.1038/nrn2733
Vasic, N., Walter, H., Sambataro, F., & Wolf, R. (2008). Aberrant functional connectivity of dorsolateral prefrontal and cingulate networks in patients with major depression during working memory processing. Psychological Medicine, 39, 977 - 987. https://doi.org/10.1017/S0033291708004443
Vizueta, N., Rudie, J., Townsend, J., Torrisi, S., Moody, T., Bookheimer, S., & Altshuler, L. (2012). Regional fMRI hypoactivation and altered functional connectivity during emotion processing in nonmedicated depressed patients with bipolar II disorder. The American Journal of Psychiatry, 169(8), 831-40 . https://doi.org/10.1176/appi.ajp.2012.11030349
Vogt B. A. (2005). Pain and emotion interactions in subregions of the cingulate gyrus. Nature Reviews. Neuroscience, 6(7), 533–544. https://doi.org/10.1038/nrn1704
Wackerhagen, C., Wüstenberg, T., Mohnke, S., Erk, S., Veer, I., Kruschwitz, J., Garbusow, M., Romund, L., Otto, K., Schweiger, J., Tost, H., Heinz, A., Meyer-Lindenberg, A., Walter, H., & Romanczuk-Seiferth, N. (2017). Influence of familial risk for depression on cortico-limbic connectivity during implicit emotional processing. Neuropsychopharmacology, 42, 1729-1738. https://doi.org/10.1038/npp.2017.59
Watkins, K. E., Vargha-Khadem, F., Ashburner, J., Passingham, R. E., Connelly, A., Friston, K. J., Frackowiak, R. S., Mishkin, M., & Gadian, D. G. (2002). MRI analysis of an inherited speech and language disorder: Structural brain abnormalities. Brain: A Journal of Neurology, 125(Pt 3), 465–478. https://doi.org/10.1093/brain/awf057
Wesson, D. W., & Wilson, D. A. (2011). Sniffing out the contributions of the olfactory tubercle to the sense of smell: Hedonics, sensory integration, and more? Neuroscience and Biobehavioral Reviews, 35(3), 655–668. https://doi.org/10.1016/j.neubiorev.2010.08.004
Witter, M. P., Wouterlood, F. G., Naber, P. A., & Van Haeften, T. (2000). Anatomical organization of the parahippocampal-hippocampal network. Annals of the New York Academy of Sciences, 911, 1–24. https://doi.org/10.1111/j.1749-6632.2000.tb06716.x
Ye, T., Peng, J., Nie, B., Gao, J., Liu, J., Li, Y., Wang, G., Ma, X., Li, K., & Shan, B. (2012). Altered functional connectivity of the dorsolateral prefrontal cortex in first-episode patients with major depressive disorder. European Journal of Radiology, 81(12), 4035-40 . https://doi.org/10.1016/j.ejrad.2011.04.058
Young, C., Chen, T., Nusslock, R., Keller, J., Schatzberg, A., & Menon, V. (2016). Anhedonia and general distress show dissociable ventromedial prefrontal cortex connectivity in major depressive disorder. Translational Psychiatry, 6. https://doi.org/10.1038/tp.2016.80
Zhang, X., Zhang, J., Wang, L., Li, R., & Zhang, W. (2016). Altered resting-state functional connectivity of the amygdala in Chinese earthquake survivors. Progress in Neuro-Psychopharmacology and Biological Psychiatry, 65, 208-214. https://doi.org/10.1016/j.pnpbp.2015.10.003
Zhou, Y., Liang, M., Jiang, T., Tian, L., Liu, Y., Liu, Z., Liu, H., & Kuang, F. (2007). Functional dysconnectivity of the dorsolateral prefrontal cortex in first-episode schizophrenia using resting-state fMRI. Neuroscience Letters, 417, 297-302. https://doi.org/10.1016/j.neulet.2007.02.081
Zhou, S., Su, S., Hong, A., Yang, C., Liu, Q., Feng, W., & Wang, Z. (2022). Abnormal functional connectivity of brain regions associated with fear network model in panic disorder. The World Journal of Biological Psychiatry, 23, 764 - 772. https://doi.org/10.1080/15622975.2022.2038389
Zhukovsky, P., Anderson, J., Coughlan, G., Mulsant, B., Cipriani, A., & Voineskos, A. (2020). Coordinate-based network mapping of brain structure in Major Depressive Disorder in younger and older adults: A systematic review and meta-analysis. The American Journal of Psychiatry, appiajp202121010088. https://doi.org/10.2139/ssrn.3730007
Zotev, V., Krueger, F., Phillips, R., Alvarez, R. P., Simmons, W. K., Bellgowan, P., Drevets, W. C., & Bodurka, J. (2011). Self-regulation of amygdala activation using real-time FMRI neurofeedback. PloS ONE, 6(9), e24522. https://doi.org/10.1371/journal.pone.0024522
Learn More
Support Our Friends

Dr. Inna Khazan's BCIA Introduction to biofeedback workshop will be offered in two parts this year.
Part 1 is entirely virtual, consisting of 20 hours (over 5 days) of live online instruction, home-study materials distributed prior to the live workshop, and written instructions for practical lab work to be completed during the week of the workshop or after its completion. Part 1 fulfills BCIA requirements for introduction to biofeedback didactic. Part 1 will take place on Zoom, November 4 - 8, 2024, 12 - 4pm EDT. Tuition is $1395.
Part 2 is optional, and consists of 14 hours (over 2 days) of in-person hands-on practical training using state-of-the-art equipment, designed to help participants be better prepared to start working with clients. Part 2 will take place in Boston on November 11 & 12, 2024, 9am-5pm EDT. Tuition is $395. (Please note that an Introduction to Biofeedback didactic (taken at any previous time, anywhere) is a pre-requisite to the hands-on training).
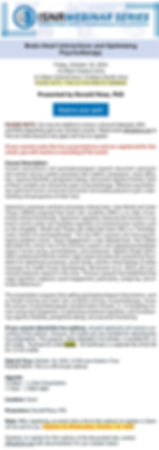
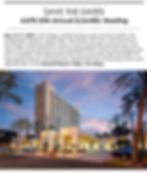