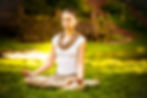
Resonance frequency (RF) assessment identifies the stimulation frequency producing the greatest amplitude heart rate oscillations in one to two sessions. We can assess the RF using slow-paced breathing (SPB) or slow-paced contraction (SPC) methods. This installment focuses on the slow-paced breathing method. We will discuss the equipment used in RF assessment and the importance of breathing assessment and training. Although we recommend using a respirometer, this does not mean you cannot measure the RF or provide effective heart rate variability (HRV) training without one. Instead, we believe that a respirometer offers assessment and training advantages.
In the third installment, we will cover RF assessment using slow-paced contraction. In the final installment, we will describe stepped and sliding protocols and their RF selection criteria. We will suggest ways to simplify RF assessment.
Click on our narrator icon to listen to this post.
Watch Dr. Inna Khazan's Heart Rate Variability: Harnessing Your Own Personal Superpower
Equipment for RF Assessment Using Slow-Paced Breathing
For publishable research, you will need an electrocardiogram (ECG) with a respirometer. We encourage using an ECG or PPG sensor with a respirometer for clinical work. Smartphone apps like Optimal HRV offer automated RF assessment using a compatible PPG sensor and their proprietary pacing display for personal training.
ECG or PPG?
Clinicians use the PPG and ECG to monitor heart rate (HR) and heart rate variability (HRV). A PPG sensor detects the pulse wave as it travels through the vascular tree.

Graphic © paulista/ Shutterstock.com.
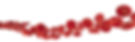
ECG sensors detect the R-spike of the QRS complex, measuring the time between successive heartbeats. A wrist placement trades ease of application for vulnerability to movement.

Graphic © arka38/Shutterstock.com.

Note. The interbeat interval (IBI), the time between successive R-spikes, is the basis for HRV time-domain, frequency-domain, and nonlinear measurements. The PPG may be less accurate than the ECG during SPB (Jan et al., 2019) and marked sympathetic nervous system (SNS) activation. Since RF protocols require breathing from 4.5-6.5 bpm and client effort may increase SNS tone, this can reduce PPG sensor accuracy. This problem is compounded if your client experiences chronic anxiety. Disorders such as anxiety are associated with peripheral vasoconstriction can interfere with detecting the pulse from the digits.
In contrast, vasoconstriction does not affect ECG detection of R-spikes from the chest, torso, or wrists (Giardino et al., 2002; Schafer & Vagedes, 2013; Shaffer & Combatalade, 2013).
Be skeptical of claims that ECG and PPG HRV measurements are correlated if the studies don't report their measurement conditions (e.g., paced breathing) and limits of agreement (i.e., allowable error; Shaffer, Meehan, & Zerr, 2020).
The Case for Using a Respirometer
Clinicians use a respirometer, a flexible sensor band that measures abdominal or thoracic movement to detect the respiratory waveform (Moss & Shaffer, 2022). Graphic © BioSource Software LLC.

Respiratory feedback serves multiple functions during RF assessment as adults breathe from 6.5 to 4.5 bpm in 0.5-bpm or smaller steps: pacing, respiration rate (RR) confirmation, and identification of dysfunctional breathing. The blue waveform in the NeXus Biotrace+ screen below represents breathing, and the red is instantaneous HR. This display reveals that the client is breathing at 5.19 bpm (top left).
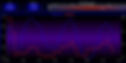
A respirometer display guides clients to breathe at prescribed rates and confirms their success. Both actions are critical because we cannot evaluate the effects of breathing at 5.5 bpm if a client actually breathed at 6 bpm. This is a serious limitation of pacing displays. Without a respirometer, we cannot immediately confirm their exact breathing rate.
An elephant in the room is that before SPB training, many clients cannot follow an animated pacing display closely at RF breathing rates (e.g., ~ 6 bpm). Graphic © Aleksandr_Kuzmin/ Shutterstock.com.
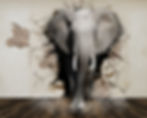
Laborde et al. (2021) reported that participants breathed 6.48-6.55 bpm when following a 6-bpm video pacing display based on Thought Technology's EZ-Air software. They did not use a respirometer to measure participants' respiration rate in real-time.
Steffen et al. (2021) cautioned:
It is important to note that the participants 6 breath per minute pacer condition were unable to consistently breathe at that rate, instead achieving an average pace of 12 breaths per minute.
Recommended Practice
At the Truman Center for Applied Psychophysiology, we repeat trials that are +/- 0.25 bpm off from the pacing display, guided by our respirometer display. Otherwise, the calculated RF could be off by +/- 0.5 bpm or worse.
Additional Advantages of Monitoring Breathing
Respiratory monitoring is also essential to identify dysfunctional breathing behaviors like apnea (breath-holding) and overbreathing (excessive exhaling of CO2), which can interfere with HRV biofeedback.
Assess Breathing Before Measuring the RF
RF measurement protocols require that clients breathe consistently at slower-than-typical rates. Breathing assessment can identify rapid breathing and dysfunctional behaviors that could interfere with RF calculation. Asthma, Generalized Anxiety Disorder, low back pain, and dysfunctional breathing behaviors like overbreathing may be associated with rapid breathing. Postpone RF assessment for adults until you successfully train them to breathe effortlessly (Peper et al., 2008) between 4.5 and 6.5 bpm.
Concerns and Contraindications
Clients who find SPB difficult may overbreathe and expel excessive CO2. If they report symptoms of feeling faint or rapid heartbeats, encourage them to take shallower and smoother breaths (Lehrer et al., 2013).
Resonance frequency assessment is contraindicated for clients whose sinus rhythm is continuously driven by a pacemaker because this device externally regulates HRV. Do not assess clients whose overbreathing compensates for increased acidity in the blood due to conditions like kidney disease. SPB could increase CO2 levels in the blood and dangerously increase acidosis (Khazan, 2013).
Practice Breathing Before RF Trials
Before starting RF trials, clinicians should provide their clients with breathing practice because the protocol requires slower-than-normal breathing rates. This precaution is crucial for clinical populations such as clients diagnosed with chronic pain. Although a healthy resting adult breathes from 12 to 20 bpm (Khazan, 2019), a RF assessment protocol instructs adults to breathe at less than half that rate.
Allow clients to practice healthy breathing from 5.5 to 6 bpm before starting RF trials. When a RR is difficult, instruct them to increase or decrease it by 0.5 bpm. For example, if a client typically breathes at 18 bpm, instruct them to decrease their breathing rate every few seconds from 18 bpm to 17.5 bpm to 17 bpm, and so forth. The Optimal HRV app provides an animated pacing display to help clients slow their breathing.
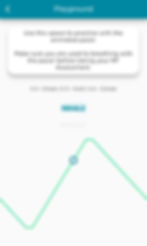
Invite your client to experiment with several inhalation-to-exhalation ratios (e.g., 1:1.5 and 1:2). Choose the easiest ratio for your client during RF assessment since slower-than-normal breathing is challenging by itself.
Whether the inhalation-to-exhalation ratio affects RF measurement remains uncertain. Within-subjects studies that utilized a respirometer and measured breathing ratios (Meehan et al., 2017; Shaffer, McCraty, & Zerr, 2014) found no difference between resting HRV metrics (e.g., HR Max-HR Min, pNN50, RMSSD, SDNN, and LF power) when participants breathed at 1:1 and 1:2 inhalation-to-exhalation ratios. Two within-subjects studies that did not directly measure breathing ratios reported increased HRV for longer exhalations than inhalations at 6 bpm (Laborde et al., 2021; Van Diest et al., 2014). During HRV biofeedback training, you can instruct clients to practice "low and slow" breathing with slightly longer exhalations than inhalations (Khazan, 2019). Although longer exhalations may not increase HRV, Lynda Thompson reports that they increase calming sensorimotor rhythm (SMR) activity (Shaffer, 2022).
Measuring the Resonance Frequency Without a Respirometer
Applications like Optimal HRV offer automated RF calculation using an animated pacer. Their 14-minute RF procedure guides clients through seven 2-minute trials with longer exhalations than inhalations: 7.0 bpm - 3.0-s inhale and 4.5-s exhale 6.5 bpm - 3.3-s inhale and 4.9-s exhale
6.0 bpm - 3.6-s inhale and 5.4-s exhale
5.5 bpm - 4.0-s inhale and 5.9-s exhale
5.0 bpm - 4.4-s inhale and 6.6-s exhale
4.5 bpm - 4.9-s inhale and 7.4-s exhale
4.0 bpm - 5.6-s inhale and 8.4-s exhale
Dr. Inna Khazan describes how to determine your RF in the following video.
The app calculates a RF using weighted criteria, including LF power, RMSSD, and HR MinMax.
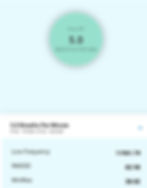
The bar chart below shows a logarithmic conversion of LF power, RMSSD, and HR MinMax values.
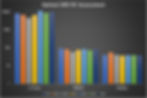
The table below shows the same data in their original units. Since Optimal HRV's algorithm assigns the greatest weight to LF power (light blue), it selected 5.0 bpm as the RF. The highest RMSSD and MinMax values were observed at 7.0 and 6.5 bpm, respectively.
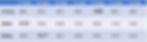
Fisher and Lehrer (2022) observed that RF criteria can point to different breathing rates, requiring clinical judgment.
There is something of an art to evaluating these metrics when they point to disparate frequencies (p. 19).
They developed a sliding protocol we will discuss in the fourth installment to simplify RF calculation and eliminate this subjectivity. Their automated RF analysis requires HR and respirometer data.
Cautions for Resonance Frequency Assessment Using Smartphone Applications
Innovative apps like Optimal HRV have made RF training more accessible and understandable. Optimal HRV allows clinicians to view client data on their Web Dashboard.
Clinicians can recommend their use by clients with the following cautions: 1. Many of these apps do not artifact IBI data, resulting in missed and extra beats that can yield invalid RF calculations. 2. The apps cannot confirm that clients breathed at the prescribed rates.
To increase the accuracy of RF calculation, we recommend the following: 1. One or more weeks of SPB practice before RF assessment. 2. Coach clients to avoid common preventable artifacts (e.g., cold, light leakage, and movement). 3. Confirm the RF during the next session using three descending 2-minute trials: RF + 0.5 bpm, RF, and RF - 0.5 bpm.
Next-session confirmation is necessary given the artifact in RF trial data and client difficulty closely following a pacing display.
Summary
We recommend an electrocardiogram (ECG) with a respirometer for publishable research and an ECG or PPG sensor with a respirometer for clinical work. Although a pacing display can guide client breathing, it cannot confirm that they consistently breathed at the required rate. A respirometer enables pacing, respiration rate (RR) confirmation, and identification of dysfunctional breathing. We recommend starting with breathing assessment and correcting dysfunctional breathing behaviors before RF assessment. If clients cannot breathe effortlessly between 4.5 and 6.5 bpm, RF assessment and subsequent HRV biofeedback training may fail. Provide "low and slow" breathing practice between 4.5 and 6.5 bpm until they can consistently follow the pacing display.
When clients use smartphone apps to calculate the RF, recognize the limitations of this approach, and consider our recommendations to increase measurement accuracy.
Glossary
apnea: breath suspension. baroreflex: baroreceptor reflex that provides negative feedback control of BP. Elevated BP activates the baroreflex to lower BP, and low BP suppresses the baroreflex to raise BP.
D2: correlation dimension estimates the minimum number of variables required to construct a system dynamics model.
electrocardiogram (ECG) sensors: electrodes that record the heart's electrical signal.
heart rate (HR) baroreflex: the closed-loop encompassing the cardiovascular control center, heart rate control system, and blood pressure control system.
high-frequency (HF) band: an HRV frequency range from 0.15-0.40 Hz representing the vagus nerve's inhibition and activation by breathing (respiratory sinus arrhythmia).
heart rate variability (HRV): the beat-to-beat changes in HR involving changes in the RR intervals between consecutive heartbeats.
HR Max – HR Min: an HRV index that calculates the average difference between the highest and lowest HRs during each respiratory cycle. Optimal HRV calls this metric, MinMax.
HRV frequency-domain measurements: metrics that quantify absolute or relative power distribution into four frequency bands, revealing the sources of HRV.
HRV nonlinear measurements: metrics that quantify the unpredictability of a time series, resulting from the complexity of the mechanisms that regulate the measured variable.
HRV time-domain measurements: metrics that quantify the total amount of HRV.
inhalation-to-exhalation ratio: the relative lengths of the inhalation and exhalation phases of the breathing cycle.
interbeat interval (IBI): the time between the peaks of successive R-spikes (initial upward deflections in the QRS complex) or pressure waves. The IBI is also called the NN (normal-to-normal) interval after removing artifacts.
limits of agreement: allowable measurement error.
low and slow breathing: effortless breathing ~ 6 bpm with slightly longer exhalations than inhalations and focused attention on the abdomen.
low-frequency (LF) band: an HRV frequency range of 0.04-0.15 Hz that may represent the influence of PNS and baroreflex activity when breathing or contracting muscles between 4.5-6.5 times a minute.
overbreathing: a mismatch between breathing rate and depth due to excessive breathing effort and subtle breathing behaviors like sighs and yawns can reduce arterial CO2.
photoplethysmographic (PPG) sensor: a photoelectric transducer that transmits and detects infrared light that passes through or is reflected off tissue to measure brief changes in blood volume and detect the pulse wave.
R-spike: the initial upward deflection of the QRS complex.
resonance: an amplification process in which an external force causes a closed-loop (negative feedback) system to oscillate with greater amplitude at its inherent resonance frequency (RF).
resonance frequency (RF): the frequency at which a negative-feedback system with a fixed delay, like the cardiovascular system, can be activated or stimulated.
resonance frequency (RF) assessment: identifying the stimulation frequency producing the greatest amplitude heart rate oscillations in one or two sessions using SPB or SPC methods.
respiratory sinus arrhythmia (RSA): the respiration-driven heart rhythm that contributes to the high frequency (HF) component of heart rate variability. Inhalation inhibits vagal nerve slowing of the heart (increasing HR), while exhalation restores vagal slowing (decreasing HR).
respirometer: a sensor that changes resistance to a current as it expands and contracts during the respiratory cycle.
RMSSD: the square root of the mean squared difference of adjacent NN intervals in milliseconds. The RMSSD is vagally-mediated HRV and the best overall measure of short-term HRV.
SD1: the standard deviation of the distance of each point from the y = x-axis that measures short-term HRV.
SD2: the standard deviation of each point from the y = x + average RR interval that measures short- and long-term HRV.
SDNN: the standard deviation of the normal (NN) sinus-initiated IBI measured in milliseconds.
sensorimotor rhythm (SMR): an EEG rhythm that ranges from 12-15 Hz and is located over the sensorimotor cortex (central sulcus). The waves are synchronous. The sensorimotor rhythm is associated with the inhibition of movement and reduced muscle tone.
slow-paced breathing (SPB): breathing at ~ 6 bpm for adults.
slow-paced contraction (SPC): wrist-core-ankle contraction with legs supported and crossed at rates of ~ 2 or 6 cpm.
time-domain measurements: quantify the total amount of heart rate variability.
triangular index (TI): a geometric measure based on 24-hour recordings, which calculates the integral of the RR interval histogram's density divided by its height.
triangular interpolation of the NN interval histogram (TINN): the baseline width of a histogram displaying NN intervals.
vagal tone: parasympathetic activity measured by the natural log of HF power when breathing at normal rates without feedback.
vascular tone (VT) baroreflex: the closed-loop encompassing the cardiovascular control center, vascular tone control system, and blood pressure control system.
References
Fisher, L. R., & Lehrer, P. M. (2022). A method for more accurate determination of resonance frequency of the cardiovascular system, and evaluation of a program to perform it. Applied Psychophysiology and Biofeedback, 47(1), 17–26. https://doi.org/10.1007/s10484-021-09524-0
Giardino, N. D., Chan, L., & Borson, S. (2004). Combined heart rate variability and pulse oximetry biofeedback for chronic obstructive pulmonary disease. Applied Psychophysiology and Biofeedback, 29(2), 121-133. https://doi.org/10.1023/b:apbi.0000026638.64386.89 Khazan, I. Z. (2013). The clinical handbook of biofeedback: A step-by-step guide for training and practice with mindfulness. John Wiley & Sons, Ltd.
Khazan, I. (2019). A guide to normal values for biofeedback. In D. Moss & F. Shaffer (eds)., Physiological recording technology and applications in biofeedback and neurofeedback. Association for Applied Psychophysiology and Biofeedback.
Laborde, S., Iskra, M., Zammit, N., Borges, U., You, M., Sevoz-Couche, C., & Dosseville, F. (2021). Slow-paced breathing: Influence of inhalation/exhalation ratio and of respiratory pauses on cardiac vagal activity. Sustainability, 13(14), 7775. http://dx.doi.org/10.3390/su13147775 Lehrer, P. (2022). My life in HRV biofeedback research. Applied Psychophysiology and Biofeedback, 1-10. https://doi.org/10.1007/s10484-022-09535-5
Lehrer, P., Kaur, K., Sharma, A., Shah, K., Huseby, R., Bhavsar, J., Sgobba, P., & Zhang, Y. (2020). Heart rate variability biofeedback improves emotional and physical health and performance: A systematic review and meta-analysis. Applied Psychophysiology and Biofeedback, 45, 109-129. https://doi.org/10.1007/s10484-020-09466-z
Lehrer, P., Vaschillo, B., Zucker, T., Graves, J., Katsamanis, M., Aviles, M., & Wamboldt, F. (2013). Protocol for heart rate variability biofeedback training. Biofeedback, 41(3), 98-109. Meehan, Z., Muesenfechter, N., Gravett, N., Watson, T., Smith, A., Shearman, S., . . . Shaffer, F. (2017). A 1:2 inhalation-to-exhalation ratio does not increase heart rate variability during 6-bpm breathing [Abstract]. Applied Psychophysiology and Biofeedback, 45, 110–111. https://doi.org/10.1007/s10484-018-9390-8
Meehan, Z. M., & Shaffer, F. (in press). Glycemic control. I. Z. Khazan, F. Shaffer, R. Lyle, D. Moss, & S. Rosenthal (Eds.). Evidence-based practice in biofeedback and neurofeedback (4th ed.).
Meehan, Z. M., & Shaffer, F. (in press). Preeclampsia. I. Z. Khazan, F. Shaffer, R. Lyle, D. Moss, & S. Rosenthal (Eds.). Evidence-based practice in biofeedback and neurofeedback (4th ed.).
Meehan, Z. M., & Shaffer, F. (in press). Adding core muscle contraction to wrist-ankle rhythmical skeletal muscle tension increases respiratory sinus arrhythmia and low-frequency power. Applied Psychophysiology and Biofeedback.
Moss, D., & Shaffer, F. (2022). A biofeedback primer. Association for Applied Psychophysiology and Biofeedback.
Peper, E., Gibney, K. H., Tylova, H., Harvey, R., & Combatalade, D. (2008). Biofeedback mastery: An experiential teaching and self-training manual. Association for Applied Psychophysiology and Biofeedback.
Schäfer, A., & Vagedes, J. (2013). How accurate is pulse rate variability as an estimate of heart rate variability? A review on studies comparing photoplethysmographic technology with an electrocardiogram. International journal of cardiology, 166(1), 15–29. https://doi.org/10.1016/j.ijcard.2012.03.119
Shaffer, F. (2022). What neuroscience has learned about the brain since grad school and why we should care. Webinar presented to the Northeast Regional Biofeedback Society. Shaffer, F., & Combatalade, D. C. (2013). Don't add or miss a beat: A guide to cleaner heart rate variability recordings. Biofeedback, 41(3), 121-130.
Shaffer, F., & Ginsberg, J. P. (2017). An overview of heart rate variability metrics and norms. Frontiers in Public Health. https://doi.org/10.3389/fpubh.2017.00258
Shaffer, F., McCraty, R., & Zerr, C. L. (2014). A healthy heart is not a metronome: An integrative review of the heart’s anatomy and heart rate variability. Frontiers in Psychology. doi:10.3389/fpsyg.2014.01040
Shaffer, F., Meehan, Z. M., & Zerr, C. L. (2020). A critical review of ultra-short-term heart rate variability norms research. Frontiers in Neuroscience.
Shaffer, F., Moss, D., & Meehan, Z. M. (2022). Rhythmic skeletal muscle tension increases heart
rate variability at 1 and 6 contractions per minute. Appl Psychophysiol Biofeedback. https://doi.org/10.1007/s10484-022-09541-7
Steffen, P. R., Austin, T., DeBarros, A., & Brown, T. (2017). The impact of resonance Frequency breathing on measures of heart rate variability, blood pressure, and mood. Frontiers in Public Health, 5, 222. https://doi.org/10.3389/fpubh.2017.00222
Steffen, P. R., Bartlett, D., Channell, R. M., Jackman, K., Cressman, M., Bills, J., & Pescatello, M. (2021). Integrating breathing techniques into psychotherapy to improve HRV: Which approach is best? Frontiers in Psychology, 12, 624254. https://doi.org/10.3389/fpsyg.2021.624254
Vaschillo, E., Lehrer, P., Rishe, N., & Konstantinov, M. (2002). Heart rate variability biofeedback as a method for assessing baroreflex function: A preliminary study of resonance in the cardiovascular system. Applied Psychophysiology and Biofeedback, 27, 1-27. https://doi.org/10.1023/A:1014587304314
Vaschillo, E. G., Vaschillo, B., Pandina, R. J., & Bates, M. E. (2011). Resonances in the cardiovascular system caused by rhythmical muscle tension. Psychophysiology, 48, 927–936. https://doi.org/10.1111/j.1469-8986.2010.01156.x
Van Diest, I., Verstappen, K., Aubert, A. E., Widjaja, D., Vansteenwegen, D., & Vlemincx, E. (2014). Inhalation/exhalation ratio modulates the effect of slow breathing on heart rate variability and relaxation. Applied psychophysiology and biofeedback, 39(3-4), 171–180. https://doi.org/10.1007/s10484-014-9253-x
Feedback

We value your feedback because we produce these posts for you. Please complete our brief survey to help us improve this service.